Raman spectroscopy requires a concentrated source of light, such as a laser, to generate a Raman signal from a sample. The characteristics of the Raman excitation laser/Raman laser primarily affect the quality of the Raman spectrum. This article discusses the lasers that are most suitable for Raman spectroscopy.
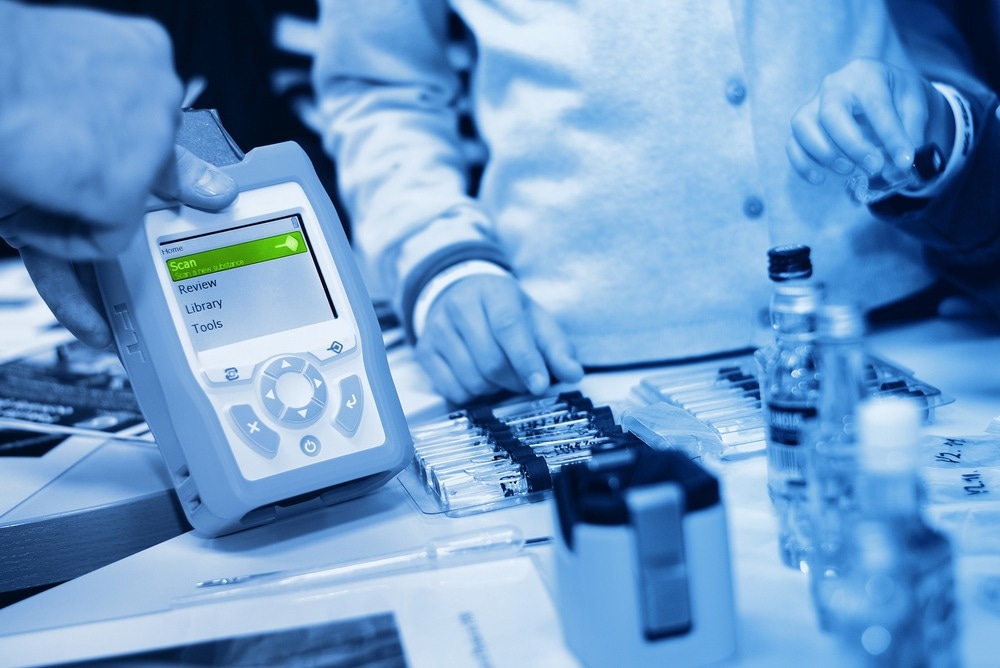
Image Credit: Forance/Shutterstock.com
The Importance of Lasers in Raman Spectroscopy
Raman spectroscopy allows a highly sensitive structural identification and easy interpretation of trace amounts of chemicals based on their distinct vibrational characteristics. Several wavelengths of light are utilized in Raman spectroscopy, including ultraviolet (UV), visible, and near-infrared (NIR).
The selection of the best laser source for a Raman spectrometer depends on several laser performance parameters, including wavelength, spectral linewidth, frequency stability, spectral purity, beam quality, output power and stability, and isolation of the laser beam. Laser linewidth, laser wavelength stability, and laser power are the most crucial among these parameters for Raman spectrometers.
The Raman laser linewidth directly impacts the Raman spectrum peak widths, while the laser wavelength must be extremely stable during Raman spectroscopy as Raman shift is a relative measurement. Additionally, adjustable laser power control is also necessary to prevent damage to highly absorbing or delicate samples due to high laser power.
Lasers for Raman Spectroscopy
Different lasers are used as the excitation source for Raman spectrometers, including 1064 nm neodymium-doped yttrium aluminum garnet lasers (Nd:YAG) lasers, 647.1 nm and 530.9 nm krypton ion lasers, 632.8 nm helium-neon (He:Ne) lasers, 630 nm and 780 nm diode lasers, and 488 nm and 514.5 nm argon ion lasers.
Although the Raman signal spatial resolution, detection sensitivity, and strength rely on the laser wavelength, which makes the use of shorter-wavelength illumination such as UV and visible more suitable compared to longer wavelengths such as NIR, fluorescence emission is a major challenge of using shorter-wavelength illumination.
Several materials emit significant fluorescence when they are excited using UV or visible illumination. This fluorescence background can heavily interfere with the weaker Raman signal, leading to difficulties in signal detection. The fluorescent effect can be reduced using a 1064 nm NIR laser in place of visible wavelength lasers.
Shifted excitation Raman difference spectroscopy (SERDS) can also avoid the influence of fluorescence. This method depends on recording two Raman spectra with two laser lines slightly shifted spectrally and then subtracting these lines from each other to eliminate the fluorescence signal.
In several materials, the impact of fluorescence can be effectively eliminated using shorter wavelengths in the UV spectral range. UV lasers excite strong fluorescence when emitted above 300 nm wavelengths. However, Raman signals can be recorded without fluorescence influence using UV lasers below 300 nm.
UV lasers are bulky and expensive, and the high energy of UV photons can damage samples, specifically solid-phase materials. Nd:YAG lasers are utilized extensively in different applications, including Raman spectroscopy. These lasers emit light with a 1064 nm wavelength, which is in the IR region of the electromagnetic spectrum.
Nd:YAG lasers are inexpensive compared to UV lasers and can be used to ensure a good balance between fluorescence background and Raman scattering efficiency. However, these lasers can damage samples and are difficult to use with several optical components.
Thus, 660–830 nm lasers are used for fluorescence suppression in Raman spectroscopy even when the Raman-scattering efficiency in this wavelength range is significantly weaker compared to the NIR wavelengths. The 785 nm has become the most commonly used laser wavelength in Raman spectroscopy due to the drawbacks of NIR and UV lasers.
This wavelength offers an excellent balance between detector efficiency, influence of fluorescence, scattering efficiency, and availability of high-quality, compact, and cost-efficient laser sources.
For instance, 785 nm diode lasers possess several advantages over Nd:YAG lasers, including longer lifetime, greater portability and compactness, higher compatibility with several optical components, and significantly reduced risk of sample damage.
Although shorter and longer wavelength lasers possess advantages and disadvantages, both types of lasers are used for Raman spectroscopy based on applications. For instance, the use of 532 nm visible lasers in the blue and green region is increasing as this illumination range is suitable for surface-enhanced Raman spectroscopy (SERS), resonance Raman experiments, and inorganic materials such as carbon fullerenes and nanotubes.
UV lasers are effective for resonance Raman spectroscopy on biomolecules such as deoxyribonucleic acid (DNA), ribonucleic acid (RNA), and proteins. Similarly, the 1064 nm NIR/Nd:YAG lasers are used in applications with extremely fluorescent materials. For instance, 1064 nm lasers have been traditionally used in Fourier-transform (FT)-Raman configurations with a single element indium gallium arsenide (InGaAs) or germanium detector to avoid interferences by fluorescence.
Solid-state Laser Sources
Currently, solid-state laser sources are increasingly being equipped with most Raman spectrometers in place of gas lasers. These compact solid-state lasers are available in all wavelength ranges used for Raman spectroscopy, can operate in industrial/harsh environments, and have an operation lifetime of several 10,000 hours, which allows users to run process-monitoring measurements/routine experiments without requiring any exchange of the laser source/servicing.
Commonly used solid-state-based continuous-wave (CW) laser sources for Raman spectroscopy can be classified as diode-pumped single-longitudinal mode lasers (DPL), single-mode diode lasers, and volume Bragg-grating (VBG) frequency-stabilized diode lasers.
DPLs can be used to achieve watt power levels at 1064 nm in the NIR and 10–50 mW power in the UV region. Additionally, several wavelengths in the blue-green-red region of the visible range, including 457 nm, 473 nm, 491 nm, 515 nm, 532 nm, 561 nm, 640 nm, and 660 nm, are available with several hundred mW of output powers.
In single-mode diode lasers, multiple wavelengths are available in the red to NIR, including 1064 nm, 980 nm, 830 nm, and 785 nm, with megahertz linewidth and output powers up to a few 100 mW. A narrow-linewidth VBG element is utilized with a diode-laser emitter in VBG frequency-stabilized diode lasers to achieve narrow-line emission at wavelengths that are unavailable as distributed Bragg reflection (DBR) or distributed feedback (DFB) sources.
To summarize, understanding the impact of laser performance on Raman spectrometers will be critical for the continued success of Raman spectroscopy in existing and new application areas. The best type of laser for Raman spectroscopy depends on the specific application requirements, with a solid-state laser/gas laser being the most suitable for applications requiring good beam quality and high power. Similarly, applications requiring illumination at a specific wavelength range must use lasers that emit light at that wavelength range.
More from AZoOptics: How is Raman Spectroscopy Used to Study Crystallography?
References and Further Reading
Gaber, D., Gaber, S., Rostron, P. (2016). Raman Spectroscopy, Review. International Journal of Engineering and Technical Research. https://www.researchgate.net/profile/Paul-Rostron/publication/309179824_Raman_Spectroscopy_a_review/links/580329fe08ae23fd1b673f34/Raman-Spectroscopy-a-review.pdf
Mulvaney, S. P., Keating, C. D. (2000). Raman Spectroscopy. Analytical Chemistry, 72(12), 145–158. https://doi.org/10.1021/a10000155
Karlsson, H., Illy, E. (2018). How to Choose a Laser: How to choose a laser for Raman spectroscopy. [Online]
Disclaimer: The views expressed here are those of the author expressed in their private capacity and do not necessarily represent the views of AZoM.com Limited T/A AZoNetwork the owner and operator of this website. This disclaimer forms part of the Terms and conditions of use of this website.