Unraveling the complex structure of biological molecules and elucidating the physics and chemistry of living organisms through a spectroscopic approach is one of the most challenging tasks encountered by modern scientists. In this context, Raman spectroscopy has become an essential tool for chemists, physicists, and biologists. By analyzing subtle molecular vibration modes, the technique enables high-sensitivity non-invasive structural studies of different water-soluble and membrane-bound biomolecules (DNA, RNA, proteins) and their interactions under physiological conditions.
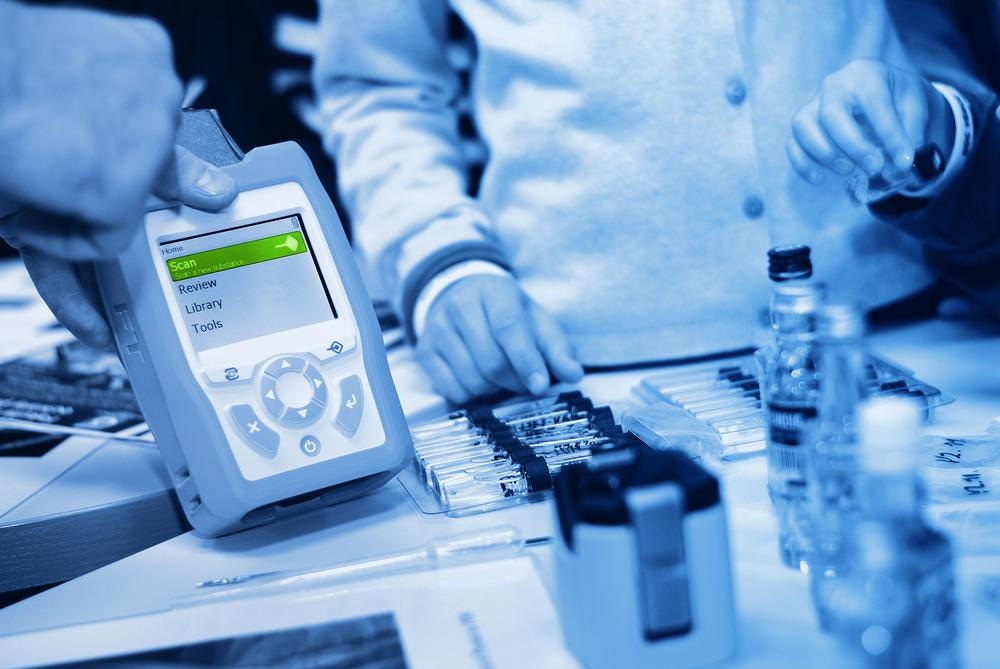
Image Credit: Forance/Shutterstock.com
The high complexity of the biological organisms, composed of biomolecules with unique functions, allows them to grow, replicate, and respond to external stimuli from the external environment. Over the past few decades, considerable efforts have been made to uncover the complex structure of biomolecules and understand their biophysics based on the common chemical and physical principles that govern all matter.
Inelastic Scattering of Photons Reveals Molecular Structures
In pursuing such a challenge, Raman spectroscopy has become one of the increasingly popular methods for investigating the unique chemical fingerprints of solid and liquid samples. Raman spectroscopy is a vibrational spectroscopic technique based on Raman scattering, a phenomenon in which incident photons lose energy via inelastic interactions with the vibrating or rotting molecules of the sample under investigation.
Since the spectrum of the scattered light depends on the molecule's unique energy levels and chemical bonds, Raman spectroscopy enables high-sensitivity chemically specific analysis of the vibration modes of molecular bonds.
The application of Raman spectroscopy to biological specimens has rapidly grown since the 1970s with the arrival of compact lasers and advanced dispersive spectrometers.
The probing photons in the laser beam couple into specific Raman vibrational or rotational modes of the molecules, losing energy in the process. The energy loss can be spectroscopically detected as a redshift in the photons' wavelength relative to the wavelength of the incident laser beam. In other words, Raman spectroscopy detects the change in energy of the Raman spectral lines relative to the energy of the excitation laser.
The Raman spectrum of complex biological molecules consists of overlapping peaks, each one corresponding to a specific molecular vibration. Assigning each of the peaks to the correct vibrational mode and picking the appropriate combination of spectral bands among the many possible ones relies on modern computational methods and comprehensive spectral databases.
Interpreting the Raman Spectrum
Most biological macromolecules consist of a small number of light elements, such as hydrogen, oxygen, carbon, and nitrogen that form covalent bonds through electron pair sharing.
This fact brings a fundamental advantage in the context of Raman spectroscopy, as the strength of covalent bonds is inversely proportional to the atomic weight of the bonded atoms. Light atoms linked by strong bonds produce more pronounced Raman shifts and clear Raman bands.
As a result, the majority of the biomolecules share only a small number of common precursors, assembled into a hierarchy of increasing structural complexity, exhibiting molecular bond vibrations that can be precisely assigned to identifiable parts of macromolecules.
Raman Spectra of Biomolecules and Cells
The high specificity of Raman spectroscopy is particularly suitable for the detection of biomolecules in cells and organisms.
Recent developments of Raman scattering-based spectroscopic and imaging techniques, such as micro-Raman, surface-enhanced and tip-enhanced Raman spectroscopy, and others, allowed researchers to probe a wide range of protein-protein and protein-lipid interactions on the micro and nanoscale. Because of its subcellular spatial resolution, Raman microscopy has been used to identify lipid, protein, and genetic materials, visualize cell structure and investigate the molecular changes in glucose, choline, cholesterol, amino acids, and other biomolecules in cell metabolism.
Raman Spectroscopy Explores DNA Structure
Raman spectroscopy enables quantitative analysis of nucleic acid conformational changes induced by the addition of binding agents such as ions, proteins, and drugs, or induced by altering experimental conditions (temperature, pH, salinity, and others).
A significant breakthrough in the detection and determination of DNA occurred with the development of surface-enhanced Raman spectroscopy. The technique has been employed as an effective and sensitive tool for detecting specific DNA sequences and can be used in next-generation sequencing tools.
Biosensing Using Raman Scattering
Biosensing applications of Raman spectroscopy focus on the detection and concentration monitoring of specific analytes in live cells and tissues. Raman spectroscopy has been actively developed as a comprehensive analytical technique. In particular, the technique has been used in non-invasive, accurate, all-optical measurements for in vivo detection of glucose concentration, enhancing the comfort of millions of diabetes patients by replacing daily blood sampling.
Deep-penetrating near-infra-red wavelength range Raman spectroscopy has been used successfully in non-invasive monitoring of brain activity behind millimeter-thick tissues and skull. Researchers have also demonstrated the use of a 785 nm excitation wavelength to monitor the concentration of neurotransmitters in simulated brain tissue via Raman spectroscopy.
Micro-Raman Imaging of Whole Cells
Combining Raman spectroscopy with modern imaging techniques enabled researchers to study intracellular and tissue distribution of compounds exhibiting strong Raman scattering, such as fatty acids, cytochromes, DNA/RNA, and various drugs. Raman spectroscopy-based imaging can also be used to discriminate between healthy and cancer cells by observing the difference in the distribution of organelles and the relative amount of various intracellular molecules.
These studies indicate that Raman spectroscopy has become an indispensable tool that can be used in all stages of biophysical and life science research, from studying fundamental properties of proteins and sensing biomolecules to clinical diagnostics. In the future, it will continue to be an essential method in fundamental research with broader applications in clinical settings for disease diagnostics.
References and Further Reading
Pezzotti, G., (2021) Raman spectroscopy in cell biology and microbiology. J. Raman Spectrosc. 1. doi.org/10.1002/jrs.6204
Orlando, A., et al. (2021) A Comprehensive Review on Raman Spectroscopy Applications. Chemosensors 9, 262. https://doi.org/10.3390/chemosensors9090262
Li, Y., et al. (2021) Review of Stimulated Raman Scattering Microscopy Techniques and Applications in the Biosciences. Adv. Biology 5, 2000184. https://doi.org/10.1002/adbi.202000184
Vlasov, A.V., et al. (2020) Raman Scattering: From Structural Biology to Medical Applications. Crystals, 10, 38. https://doi.org/10.3390/cryst10010038
Kang, J. W., et al. (2020) Direct Observation of glucose fingerprint using in vivo Raman spectroscopy. Science Advances 6, aay5206. https://doi.org/10.1126/sciadv.aay5206
Disclaimer: The views expressed here are those of the author expressed in their private capacity and do not necessarily represent the views of AZoM.com Limited T/A AZoNetwork the owner and operator of this website. This disclaimer forms part of the Terms and conditions of use of this website.