Photoelectron spectroscopy involves the detection of the kinetic energies of photoelectrons emitted following an ionization event. If it is assumed that the emitted photoelectrons do not undergo any collisional event post-ionization, the kinetic energy of the detected electron should be equal to the binding energy of the orbital it was ejected from.
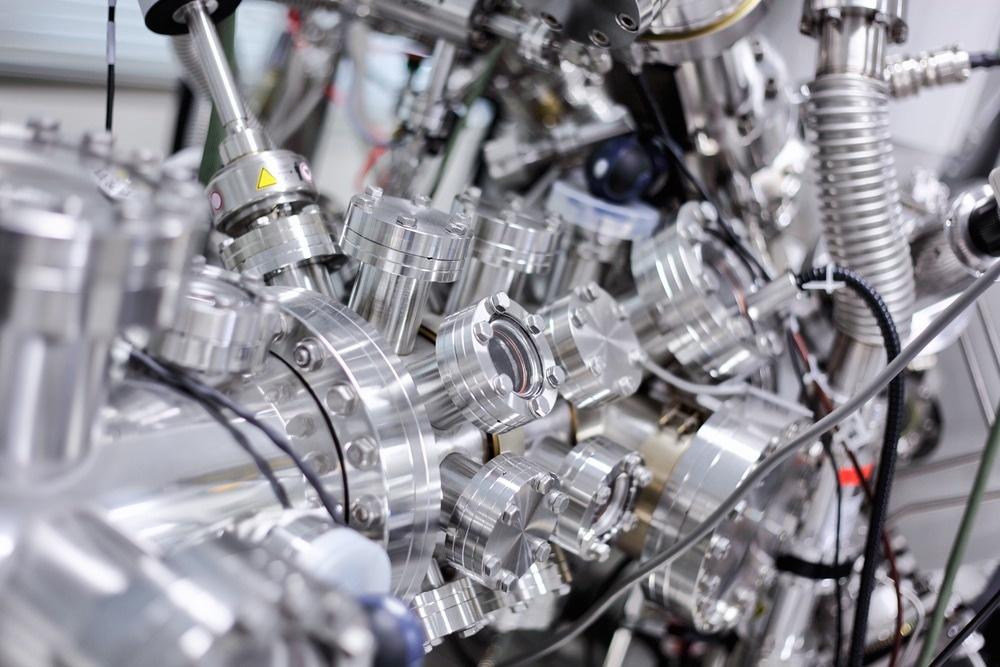
Image Credit: Alexander Gatsenko/Shutterstock.com
Information on binding energies provides insight into the electronic structure of an atom, molecule or material and can be used to understand how the species may potentially interact with light or other chemical species. Photoelectron spectroscopy has become a routine tool in industrial applications and may advance research areas, including the profiling of 2D materials and machines.1,2
Implementation
A typical photoelectron spectrometer will consist of an ionization source, a sample delivery system and a detector. Generally, photoelectron spectrometers use high-vacuum conditions to ensure that the emitted photoelectron does not lose any energy through collisions with atmospheric species. Some methods use differential pumping systems to make measurements on samples under environmental conditions akin to what they would be in situ, known as ambient pressure X-ray photoelectron spectroscopy.3
Ionization Sources
The characteristics of the ionization source in photoelectron spectroscopy are incredibly important and determine what kind of chemical information can be probed. Firstly, the source must be sufficiently high energy to be able to eject a photoelectron from the sample. Many rare gases have ionization energies above 10 eV and small, aromatic molecules are around 9 eV. It can be challenging to generate sufficiently energetic photons to ionize the target in a single step.
Helium (I) lamps, which emit at 21.2 eV have been a popular ionization source for photoelectron experiments but multiphoton ionization approaches have also proved successful.4 Multiphoton ionization relies on the absorption of multiple photons to cause ionization and is best achieved with intense light sources such as laser systems.
Another alternative ionization source has been advanced light sources such as synchrotrons. For static photoelectron measurements, synchrotrons represent an ideal light source as they have a high photon dose, a high degree of energy tunability, and sources available to generate photon energies from the UV to the hard X-ray regime.5
Varying the incident photon energy in a photoelectron spectroscopy experiment changes the type of chemical information available. While the valence band information obtained with lower energy visible or UV photons is particularly useful for determining the optical properties of molecules or materials, electron spectroscopy for chemical analysis (ESCA) relies on ionization of the tightly bound core electrons requiring X-ray radiation.
ESCA and X-ray photoelectron spectroscopy identify the chemical composition of materials but also provide information on the local bonding environment of the elements. The binding energies of the core electrons are very element-specific. While there are small changes in the exact energy depending on the local environment, the energies are typically specific enough for the elemental analysis to be performed. This is why ESCA is now such a commonly used approach in many areas of science, including art preservation and historical research.6
Detectors
The detection scheme used in a photoelectron experiment is critical to achieving the kinetic energy resolution and determining the efficiency with which ionization events can be detected. Many photoelectron instrument use time-of-flight detection schemes, where the flight time of the electron is used to determine its kinetic energy. Some schemes use electron imaging to look at observables such as the photoelectron angular distributions which can provide additional information on the nature of the orbital the photoelectron was ejected from.7
Typical types of detectors used include magnetic bottles, hemispherical analyzers and, for imaging, cameras coupled to imaging plate stacks. Each has its advantages and disadvantages and the optimal choice of the detector depends on the type of samples being used, the photon energy range, and the desired resolution. For example, magnetic bottles offer outstanding collection efficiencies and hemispherical analyzers can be used for both spectroscopy and imaging approaches, often with excellent energy resolution.
Future Outlook
While both lab and facility-based photoelectron spectroscopy is now a routine experiment, there are still many active developments in the field. With the increasing availability of short-pulse laser systems and free-electron laser facilities, that can generate very bright pulses of X-ray radiation, time-resolved photoelectron measurements are becoming increasingly commonplace. Such measurements make it possible to visualize in real-time what processes happen when the sample interacts with electromagnetic radiation.
Volatile liquids have historically posed a problem for photoelectron measurements, as they are incompatible with the high vacuum requirements for detecting unperturbed electrons. However, there are now commercial solutions available for forming liquid jets in a vacuum to perform photoelectron studies on surface and bulk materials.9
Technologies such as high harmonic generation make it possible to achieve higher energy photons in the laboratory environment, often still with excellent time resolution. They also enable new types of time-resolved photoelectron experiments to be performed.
References and Further Reading
- Morgan, D. J. (2019). Imaging XPS for industrial applications. Journal of Electron Spectroscopy and Related Phenomena, 231, 109–117. https://doi.org/10.1016/j.elspec.2017.12.008
- Addou, R., & Wallace, R. M. (2019). Using photoelectron spectroscopy in the integration of 2D materials for advanced devices. Journal of Electron Spectroscopy and Related Phenomena, 231, 94–103. https://doi.org/10.1016/j.elspec.2018.01.006
- Arble, C., Jia, M., & Newberg, J. T. (2018). Lab-based ambient pressure X-ray photoelectron spectroscopy from past to present. Surface Science Reports, 73(2), 37–57. https://doi.org/10.1016/j.surfrep.2018.02.002
- Anderson, S. L., Rider, D. M., & Zare, R. N. (1982). Multiphoton ionization photoelectron spectroscopy: a new method for determining vibrational structure of molecular ions. Chemical Physics Letters, 93(1), 11–15. https://doi.org/10.1016/0009-2614(82)85045-8
- Suga, S., & Sekiyama, A. (2016). Photoelectron spectroscopy. Springer-Verlag Berlin An. https://doi.org/10.1007/978-3-030-64073-6
- Pintori, G., & Cattaruzza, E. (2021). XPS/ESCA on glass surfaces: A useful tool for ancient and modern materials. Optical Materials: X, 100108. https://doi.org/10.1016/j.omx.2021.100108
- Reid, K. L. (1998). Photoelectron Angular Distributions. Annual Review of Physical Chemistry, 2003(54), 397–424. http://dx.doi.org/10.1016/j.jaci.2012.05.050
- Stolow, A., Bragg, A. E., & Neumark, D. M. (2004). Femtosecond time-resolved photoelectron spectroscopy. Chemical reviews, 104(4), 1719-1758. https://doi.org/10.1021/cr020683w
- Buchner, F., Lübcke, A., Heine, N., & Schultz, T. (2010). Time-resolved photoelectron spectroscopy of liquids. Review of Scientific Instruments, 81(11), 113107. https://doi.org/10.1063/1.3499240
Disclaimer: The views expressed here are those of the author expressed in their private capacity and do not necessarily represent the views of AZoM.com Limited T/A AZoNetwork the owner and operator of this website. This disclaimer forms part of the Terms and conditions of use of this website.