Enzymology is the study of the structure and dynamics of enzymes, including their interactions with other species. Enzymes are highly efficient biological catalysts and so there is a great scientific drive to understand what makes them highly suited to creating artificial mimics that can achieve similar catalytic properties.1
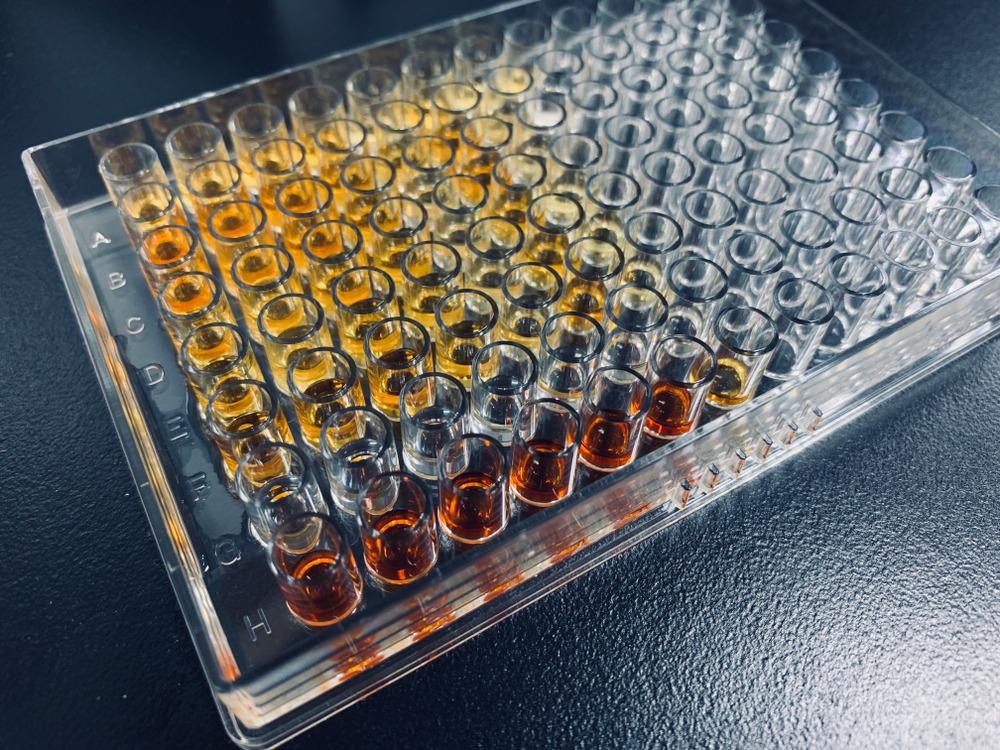
Image Credit: Buntan/Shutterstock.com
Enzymes tend to be large biological species, with many composed of different proteins. Some enzymes also require the presence of additional chemical species, called cofactors, to perform their catalytic tasks.2
Most enzymes have specifically shaped active sites that help them selectively bind the substrates for the reactions they catalyze. This selectivity makes many enzymatic species highly selective – some enzymes can only catalyze one specific chemical reaction.
From a chemical perspective, the large number of atoms present in an enzyme makes them challenging to study at a mechanistic level. However, one of the most fruitful approaches for recovering detailed information on the intermolecular interactions between enzymes and their substrates as well as the timescales for enzymatic reactions has been combining different spectroscopic techniques to construct a picture of what is happening at the atomistic level.
Spectroscopy Toolbox
Spectroscopy encompasses a highly diverse range of experimental techniques. The advantage of the wealth of different methods available is that each type of spectroscopy is sensitive to different experimental observables. For example, Raman and infrared spectroscopy are both typically sensitive to molecular vibrations but as the type of interaction between the electromagnetic radiation and the sample measurement is different, vibrations that can be detected with one technique may not be observed in the other and vice versa.
For enzymology, infrared spectroscopy has become one popular method, particularly for monitoring binding kinetics and turnover rates. The sensitivity of infrared spectroscopy to vibrational frequencies, that are in turn indicative of the presence of particular chemical functional groups, makes it ideal for identifying changes between reactants and products.3
Infrared spectroscopies can also be extended to the time domain. While it has been possible to record infrared spectra over a matter of minutes, which is sufficient to track product formation in many enzymatic reactions, the fastest enzymes can catalyze reactions on the order of picoseconds and so required the use of ultrafast laser spectroscopies or advanced electronic synchronization schemes to probe.4
Such probing methods can also be combined with other reaction initialization techniques, sometimes known as ‘jump’ methods, where a sudden change in the pH, temperature, or another variable, triggers the enzymatic reaction of interest.4 The higher time resolution makes it possible to not just capture what happens in faster enzymatic processes but also to potentially identify short-lived reactive complexes or intermediates that would not be detectable using traditional steady-state approaches.
Other Probes
Other common tools to identify both enzyme composition and recover dynamical information are nuclear magnetic resonance (NMR) spectroscopy approaches. While the achievable time-resolution with NMR may not be as effective as ultrafast laser techniques, NMR still remains a sensitive probe of the enzyme configuration and can be used to recover some information on the binding and reactive geometries of the enzyme.5
NMR measurements are sensitive to a particular nucleus, which can be used to achieve elemental selectivity with the technique. Advances in the isotope labeling of enzymes have also made it possible to use NMR to specifically look at the dynamical behavior of certain sites.5 This can be particularly useful for understanding how the substrate binding occurs and which interactions give rise to the enzymatic selectivity.
Metalloenzymes
Approximately a third of all enzymes contain a metal species and these so-called metalloenzymes have been of particular interest for the creation of artificial biomimics. Many of the traditional chemical catalysts used involve metal-containing complexes and so trying to emulate the catalytic function of metalloenzymes has been an obvious step in catalysis research.6
The presence of a metal atom or ion in the enzyme also has a significant influence on what types of spectroscopic techniques can be used to probe the behavior of the metalloenzyme. The metal sites are often very important for the reactivity of the metalloenzyme and properties such as the spin state and oxidation state of the metal center play an important role in the catalytic cycles.7
X-ray spectroscopies are ideally suited for selective probing of the metal sites as their element selectivity means the properties of the metal site can be observed without too much interference of signals from the rest of the protein structure. Such methods have also been extended to the time domain to recover dynamical information on enzymatic behavior.8
Electron paramagnetic resonance (EPR) spectroscopy is another method well-suited to the study of metalloenzymes where the metal center has unpaired electrons.9 This technique can also be used to look at the metalloenzyme itself and how it interacts with other metallic species.
Spectroscopy is an invaluable tool in enzymology due to its ability to capture information on nearly the complete catalytic cycle. Combining tools makes it possible to detect both the behavior of the enzyme and its substrate and has become invaluable in our understanding of molecular biochemistry and for the creation of new enzymatic mimics.
References and Further Reading
- Marsh, E. N. G. (2016). Whither enzymology in the twenty first century? Frontiers in Chemistry, 4, 1–5. https://doi.org/10.3389/fchem.2016.00020
- Robinson, P. K. (2015). Enzymes: principles and biotechnological applications. Essays in Biochemistry, 59, 1–41. https://doi.org/10.1042/BSE0590001
- Kumar, S., & Barth, A. (2010). Following enzyme activity with infrared spectroscopy. Sensors, 10(4), 2626–2637. https://doi.org/10.3390/s100402626
- Callender, R., & Dyer, R. B. (2006). Advances in time-resolved approaches to characterize the dynamical nature of enzymatic catalysis. Chemical Reviews, 106(8), 3031–3042. https://doi.org/10.1021/cr050284b
- Palmer, A. G. (2015). Enzyme dynamics from NMR spectroscopy. Accounts of Chemical Research, 48(2), 457–465. https://doi.org/10.1021/ar500340a
- Wittwer, M., Markel, U., Schiffels, J., Okuda, J., Sauer, D. F., & Schwaneberg, U. (2021). Engineering and emerging applications of artificial metalloenzymes with whole cells. Nature Catalysis, 4(10), 814–827. https://doi.org/10.1038/s41929-021-00673-3
- Lothian, A., Hare, D. J., Grimm, R., Ryan, T. M., Masters, C. L., & Roberts, B. R. (2013). Metalloproteomics: Principles, challenges, and applications to neurodegeneration. Frontiers in Aging Neuroscience, 5(JUL), 1–7. https://doi.org/10.3389/fnagi.2013.00035
- Kern, J., Schoenlein, R. W., & Wernet, P. (2021). Using X-ray free-electron lasers for spectroscopy of molecular catalysts and metalloenzymes. Nature Reviews Physics, 3(April). https://doi.org/10.1038/s42254-021-00289-3
- Eisermann, J., Seif-Eddine, M., & Roessler, M. M. (2021). Insights into metalloproteins and metallodrugs from electron paramagnetic resonance spectroscopy. Current Opinion in Chemical Biology, 61, 114–122. https://doi.org/10.1016/j.cbpa.2020.11.005
Disclaimer: The views expressed here are those of the author expressed in their private capacity and do not necessarily represent the views of AZoM.com Limited T/A AZoNetwork the owner and operator of this website. This disclaimer forms part of the Terms and conditions of use of this website.