Quantum materials often exhibit exotic physical properties directly tied to the quantum mechanical nature of electrons, such as superconductivity and charge density waves at low temperatures (∼93 K and lower). At such extreme temperature conditions, the ability of the cryogenic transmission electron microscopy to image heterogeneous and complex materials, where composition, physical, and electronic structure need to be analyzed at an atomic scale, becomes critical when studying quantum phenomena.
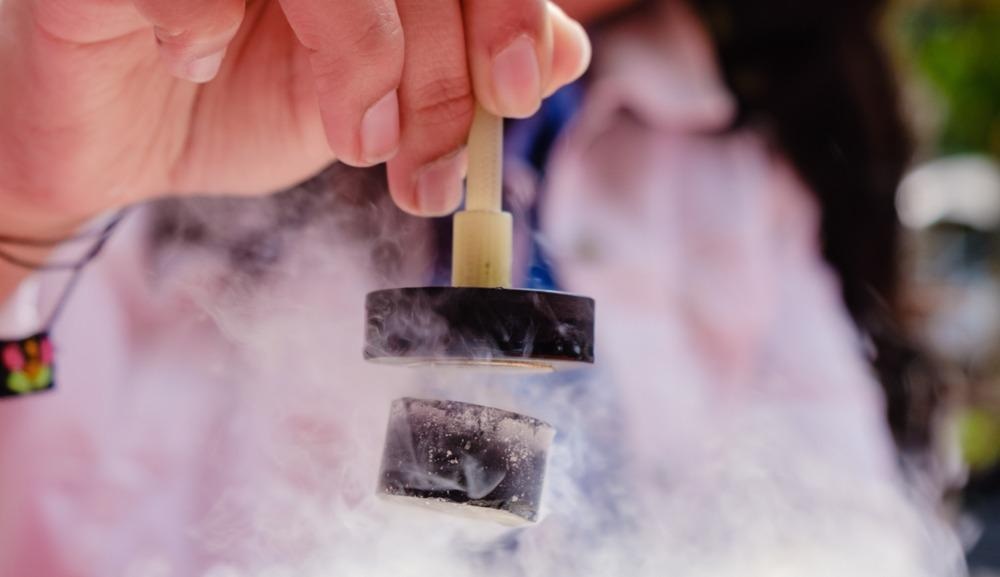
Image Credit: michelmond/Shutterstock.com
At a fundamental level, quantum mechanics defines material properties by governing how atoms bond and electrons interact. In many cases, these quantum effects can be described by classical physics at the macroscopic level.
With the discovery of superconductivity more than a century ago, a new class of materials and phenomena emerged, whose fundamental properties cannot be described in terms of classical condensed-matter physics. Instead, it requires examination through the prism of exotic quantum-mechanical effects, such as quantum coherence and quantum entanglement.
Today, the concept of quantum materials describes a broad range of materials where quantum mechanics manifests itself on a macroscopic level leading to strong electron-electron and electron-lattice interactions or some other type of electron ordering within the material bulk.
This concept attracts interest from diverse sectors of science and engineering, including condensed-matter and low-temperature physics, materials science, and quantum computing.
Unique Insides into the Quantum World at Low Temperatures
Quantum phenomena such as superconductivity and quantum information processing are more easily and commonly studied at low temperatures (from ∼93 K down to a few mK). Often, these exotic properties related to the quantum mechanical nature of electrons only exist at low temperatures.
The majority of the low-temperature studies involving quantum materials are performed by scanning tunneling microscopy (STM) or spectroscopy. These techniques provide atomic-resolution imaging and electronic structure information with a spectroscopic resolution of around 11 μeV and can elucidate quantum phenomena at temperatures down to 10 mK.
However, scanning probe techniques only provide information relevant to the sample surface, and cannot reveal structural information about internal defects or the three-dimensional microstructure.
Taking Structural Studies into a New Era
The latest advancements in cryogenic transmission electron microscopy (cryo-TEM) had a profound impact on life sciences, recognized by awarding the 2017 Nobel Prize in Chemistry for the technique's development.
Modern automated cryo-TEMs enable freezing and stabilizing fragile biomolecules for near atomic-resolution imaging in their native states, revolutionizing the field of structural biology in the last decade.
A major weakness of modern cryo-TEMs used in materials science is the sample holder design, where the specimen is thermally and mechanically coupled to a cryogenic coolant, resulting in resolution tradeoffs even on the most stable cryo-TEM platforms (compared to standard room temperature conditions).
Until recently, these instrument limitations, causing thermal drift and coupling of external vibrations, precluded high-resolution imaging of sub-picometer lattice variations in real space and in real-time.
Ongoing developments, both by instrument manufacturers and academic research groups, of mechanically decoupled cryo-TEM sample holders with improved stability (extremely low drift and vibration levels), promise to enable sub-atomic resolution imaging and electron spectroscopy at continuously variable temperatures in the 77 K–1000 K range.
Cryo-TEM for Quantum Science
A striking illustration of the potential of the improved cryo-TEMs to image material structures directly in real space with extremely high resolution is the visualization of emergent charge density phases in a manganite crystal.
Researchers from Cornel University in New York, led by Prof. Lena Kourkoutis, compared picometer-scale lattice distortions in ultra-high-resolution cryo-TEM at room temperature and cryogenic conditions (∼93 K). These results could help to explain how charge-ordering impacts other coupled electronic states in quantum materials such as cuprates and manganites (family of superconducting and magnetic materials, respectively).
Spatially-resolved spectroscopic characterization via cryo-TEM electron energy loss spectroscopy (EELS) can provide additional valuable insights about the local changes in chemical bonding and valence state within the atomic lattice of the quantum materials. A research group from the Massachusetts Institute of Technology in the USA studied the interface between iron selenide (FeSe) thin films and SrTiO3 substrate by low-temperature EELS at 10K to reveal the mechanism leading to enhanced superconductivity in the thin FeSe film (compared to bulk FeSe).
Real-space structural imaging by cryo-TEM also permits to follow phase transition pathways in quantum materials that cannot be visualized through diffraction or surface imaging techniques. A research group at the University of Maryland in the USA studied the ferroelectric domain structure of tin telluride (SnTe) nanowires at a cryogenic temperature of 25 K. The observed ferroelectric transition at such low temperatures was essential for the understanding of the superconducting properties of these nanowires.
Future Prospects for Cryo-TEM
Researchers and instrument developers believe that further improvement of cryo-TEM's spatial resolution is of critical importance for direct observations of quantum phenomena. For example, atomic lattice distortions on the level of picometers can characterize an electronic phase transformation.
Reaching this goal is not limited by fundamental physics, but rather by engineering and instrumentation challenges, which the scientists are working to overcome. Designing advanced cryo-holders with an extended temperature range below 10 K, while permitting multi-axis tilt and rotation of the specimens, will be necessary to access many emerging properties in the next generation of quantum materials.
References and Further Reading
J. L. Hart and J. J. Cha (2021) Seeing Quantum Materials with Cryogenic Transmission Electron Microscopy. Nano Letters 21 (13), 5449-5452. Available at: https://doi.org/10.1021/acs.nanolett.1c02146
C. J. Trimble, et al. (2021) Josephson Detection of Time Reversal Symmetry Broken Superconductivity in SnTe Nanowires. npj Quantum Mater. 6, 61. Available at: https://doi.org/10.1038/s41535-021-00359-w
Y. Li, et al. (2020) Opportunities for Cryogenic Electron Microscopy in Materials Science and Nanoscience. ACS Nano 14 (8), 9263-9276. Available at: https://doi.org/10.1021/acsnano.0c05020
A. M. Minor, et al. (2019) Cryogenic electron microscopy for quantum science. MRS Bulletin 44, 961–966. Available at: https://doi.org/10.1557/mrs.2019.288
D. W. McComb, et al. (2019) Cryogenic transmission electron microscopy for materials research. MRS Bulletin 44, 924–928. Available at: https://doi.org/10.1557/mrs.2019.283
I. El Baggari, et al. (2018) Cryo-STEM imaging of incommensurate charge order. Proc. Nat. Acad. Sci. 115 (7) 1445-1450. Available at: https://doi.org/10.1073/pnas.1714901115
W. Zhao, et al. (2018) Direct imaging of electron transfer and its influence on superconducting pairing at FeSe/SrTiO3 interface. Science Advances, 4, 3, eaao2682. Available at: https://doi.org/10.1126/sciadv.aao2682
Disclaimer: The views expressed here are those of the author expressed in their private capacity and do not necessarily represent the views of AZoM.com Limited T/A AZoNetwork the owner and operator of this website. This disclaimer forms part of the Terms and conditions of use of this website.