In an article published in Energies, researchers presented a novel approach to improve the optoelectrical properties of silicon (Si), including optical conductivity and bandgap, by applying the direct ultrafast pulsed nanostructure formation (DUPNF) technique.
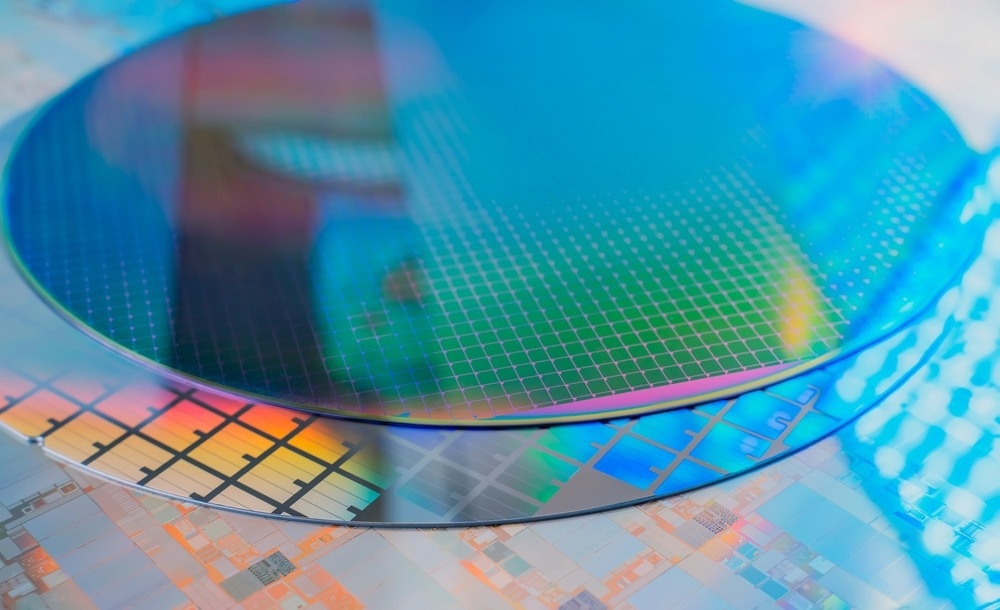
Study: Synthesis of 3D Nanonetwork Si Structures via Direct Ultrafast Pulsed Nanostructure Formation TechniqueImage Credit: asharkyu/Shutterstock.com
The 3D nanonetwork Si structures were created by varying the power and frequency of the high-intensity picosecond laser pulses. The optical characteristics of the 3D nanonetwork Si structures were investigated using optical spectroscopy in the near-infrared (NIR) and visible (VIS) regions.
The energy-dispersive X-ray (EDX) and scanning electron microscopy (SEM) imaging techniques analyzed the structural properties of the Si structures. Varying the laser processing frequency and power led to a change in the structural properties and bandgap of the 3D nanonetwork Si structures.
The development of the 3D nanonetwork Si structures and the modification in their characteristics were visible as the frequency and power of the laser pulses were increased. Compared to bulk silicon, the critical breakdown field of the Si structures was more significant.
Benefits of Nanostructures
Nanomaterials have gained popularity as a result of advances in technology and nanoscience. Material characteristics improve at the nanoscale and when the scale is reduced, the size decreases, enabling the material to be utilized in compact devices.
Nanomaterials are essential as the current global technology demands smaller devices without reduced functionality.
Silicon, one of the most extensively available semiconductors, has several interesting applications in electronics, sensors, energy generation, and biomedical systems. However, the use of silicon in multiple high-power applications is restricted by its relatively low indirect bandgap of 1.12 eV. Its low critical breakdown field, at 0.2 MV/cm, also acts as a constraint in its high-power applications.
The shift to nanomaterials has enabled the alteration of the characteristics of materials. It has been observed that nanomaterials have unique features contrary to bulk materials. The synthesis of the 3D nanonetwork Si structures utilizing various methods, including spray pyrolysis, chemical vapor deposition, sol-gel technology, and laser deposition, has been extensively studied and published by multiple scholars.
However, as some of these procedures involve an expensive setup and preparation environment, research has been shifting toward newer innovative synthesis techniques. A unique approach for the environmentally friendly and economical synthesis of Si structures in ambient settings has been demonstrated.
The direct ultrafast pulsed nanostructure formation method provided a simple, one-step process for creating Si structures with predetermined optical characteristics.
The changes in the characteristics of the 3D nanonetwork Si structures synthesized via direct ultrafast pulsed nanostructure formation were reported as a function of the laser parameters such as power and frequency.
The amount of energy used for ablation and the laser's power significantly impacted the formation of the nanostructures. A plasma layer was eventually formed due to the laser's interaction with the material and the substrate's energy absorption.
The interaction between the laser and the material was controlled with ultrashort laser pulses, which also helped reduce the material damage. The ultra-short pulses aided the surface disintegration into fine particles deposited over the sample.
The power and frequency parameters varied to determine the effects on the laser's pulse energy and duration.
Synthesizing the Enhanced Si Nanostructures
Twenty-micrometer thick silicon n-type (Si-100) wafers were utilized for the experiment. For synthesizing the nanostructures, silicon was irradiated with a pulsed fiber laser at a pulse duration of 150 ps and a scan speed of 100 mm/s when applying the direct ultrafast pulsed nanostructure formation technique.
By varying the laser's power and frequency (1200, 1000, 800, and 600 kHz), four samples were generated, which were named the "S" and "A" sets. The "A" samples (constant power) had fluctuating frequency and constant power at 15 W.
The "S" samples, with constant pulse energy, had fluctuating frequency and variable power at 10, 13.3, 16.7, and 20 W with continuous energy (110 mJ).
The experimental setup for the direct ultrafast pulsed nanostructure formation technique consisted of a sample holder, spectrometer, light source, and optical cables. Optical tests were performed on the samples by applying light spectroscopy, and the reflectance spectra were obtained and examined. The SpectraSuite software was used to record and examine the reflectance curve and data.
The samples were first examined via SEMl; the sample S4 contained more 3D nanonetwork structures. In the region where the direct ultrafast pulsed nanostructure formation approach was used, the S1 to S3 samples had an increased brightness.
A similar tendency was also present in the A samples. A1 to A4 demonstrated that the samples' colors became lighter and brighter. When examined by the unaided human eye, the ablated area of the S2 and S3 samples was comparable to the A4 sample.
Overall, the samples displayed an increase in their bandgap after applying the direct ultrafast pulsed nanostructure formation technique due to the formation of more nanofibers which enhanced the material's bandgap.
The number of overlaps between distinct energy levels in the material's band structure decreased as the number of produced nanoparticles improved, raising the material's bandgap.
The samples' optical conductivity was also assessed using the reflectance information gathered by light spectroscopy. The relation between the material's bandgap and refractive index affects the optical conductivity. Consequently, the optical conductivity decreased as the samples' bandgap increased after applying the direct ultrafast pulsed nanostructure formation technique.
Novel Approach Enhances Silicon’s Optoelectrical Characteristics
A novel approach of employing the direct ultrafast pulsed nanostructure formation technique to generate 3D nanonetwork Si structures with enhanced characteristics was proposed in this paper.
Various 3D nanonetwork Si structures were formed by changing the laser parameters, including power and frequency. Spectroscopic methods were used to determine the characteristics of the 3D nanonetwork Si structures. The material's bandgap was finally improved, and the variation in pulse duration and pulse energy was used to measure the bandgap's change.
The relation between the bandgap and refractive index is crucial for determining the material's other optical characteristics. It was observed that as the band gap expanded, the refractive index decreased. Other optical parameters were also analyzed, and it was discovered that while the samples' dielectric constant increased, their optical conductivity decreased.
The critical breakdown field was also calculated, and a critical field resembling the gallium-arsenide (GaAs) was obtained for the nanostructure samples.
The findings of this work contribute to potential ways to enhance the optoelectrical characteristics of semiconductors using the simple, quick, direct, and ultrafast pulsed nanostructure formation technique.
Reference
N.S. Jamwal, A. Kiani, 2022. Synthesis of 3D Nanonetwork Si Structures via Direct Ultrafast Pulsed Nanostructure Formation Technique. Energies. https://www.mdpi.com/1996-1073/15/16/6005
Disclaimer: The views expressed here are those of the author expressed in their private capacity and do not necessarily represent the views of AZoM.com Limited T/A AZoNetwork the owner and operator of this website. This disclaimer forms part of the Terms and conditions of use of this website.