Atomic force microscopy (AFM) is a powerful tool in microscopy. It is used to obtain high-resolution nanoscale images to gain a deeper comprehension of the properties of matter. AFM has, therefore, been vital to the advancement of biomedical research.
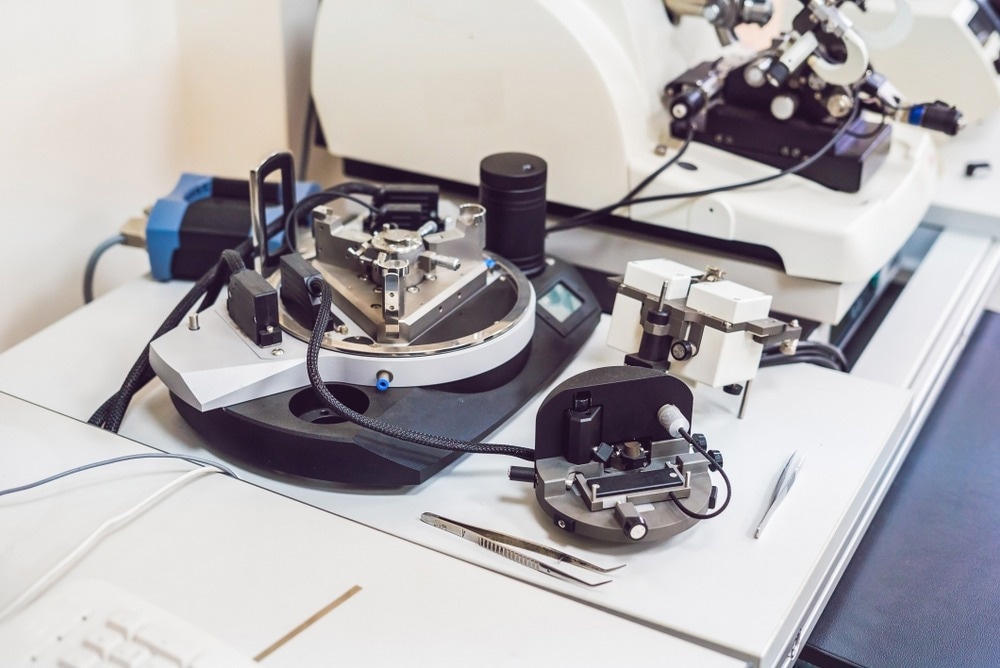
Image Credit: Elizaveta Galitckaia/Shutterstock.com
In 1986, AFM emerged from a ground-breaking experiment. Prior to this, IMB-Research's Gerd Binnig and Heinrich Rohrer were awarded a Nobel Prize for developing the scanning tunneling microscope (STM). However, the STM was limited to imaging semiconductors or metallic materials.
To address this, Binnig created the AFM in collaboration with Christoph Gerber and Calvin Quate. Unlike the STM, the AFM can image any material with exceptional resolution, surpassing the STM's capabilities. Remarkable resolutions of a million times smaller than a human hair can be resolved in AFM images.
Working Principle of AFM
A sharp-tipped cantilever is the first of four essential components in an AFM. The back of the cantilever is coated with a reflective material, causing it to reflect light similar to a mirror.
A photodetector, which is extremely sensitive to capturing photons from laser beams, gathers the reflected light once a laser is pointed toward the rear of the cantilever.
An electric controller precisely scans the cantilever, laser, and detector system. The sample, secured on a translation stage, can also be moved beneath the microscope. Figure 1 illustrates a schematic diagram of an AFM.
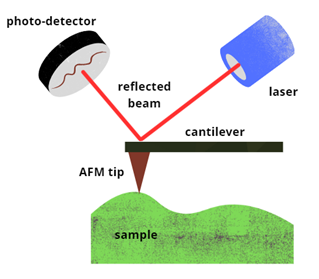
Figure 1: Schematic diagram of an Atomic Force Microscope (AFM). Image Credit: Ilamaran Sivarajah
The cantilever compresses and stretches based on the force applied between the tip and the sample. The photodetector detects changes in the position of the reflected laser beam in proportion to the movement of the cantilever. Scanning across the material's surface produces a detailed topographical image of the sample.
Electrostatic forces between charges on the sample's surface and the tip represent one type of force encountered between the AFM tip and the sample. Other observed forces include van der Waals forces, mechanical contact forces, capillary forces, magnetic forces, Casimir forces, and forces resulting from chemical bonding. Various modes of operation are available for the AFM to suit the type of force being measured.
The AFM hardware offers multiple imaging modes for extracting different types of sample data. They can be broadly divided into contact modes, non-contact modes, and tapping modes, depending on the dynamics of the probe-sample interaction.
Careful selection of AFM modes of operation is essential to ensure optimal imaging performance. Biological samples, being pliable and prone to rupture, can experience deformation and severe image distortions due to the friction force generated during basic AFM contact mode imaging.
This problem is particularly pronounced with soft biomedical samples, which are more susceptible to damage. In such cases, tapping modes between the probe tip and the sample are advantageous.
Consideration of the imaging mode and operating environment is crucial when selecting the appropriate cantilever probe. Key factors include tip geometry, cantilever stiffness, and reflection efficiency.
AFM in Biomedical Research
AFM has played a substantial role in the advancement of biomedical research, particularly in areas such as cancer screening and diagnosis. The utilization of AFM in analyzing individual cancer cells offers two anticipated benefits.
Firstly, early cancer detection relies on observing changes and distinctions between individual cells, enabling early diagnosis and therapy based on the distinction between cancerous and non-cancerous cells.
Secondly, AFM facilitates the investigation of cancer cell composition, capabilities, dissemination processes, cell-cell interactions, and the effects of anti-cancer medications.
For instance, AFM has revealed differences in white blood cell surface morphology between healthy individuals and those with chronic leukemia. By comparing cells to tumor cells from the same source, AFM can distinguish malignant from non-malignant cells and illustrate notable changes in cell membrane shape between normal and tumor cells. This offers a dependable supplementary foundation for differential and clinical pathology diagnoses.
The mechanical strength of cells influences tissue homeostasis, cell development, division, migration, and the epithelial-mesenchymal transition. AFM research has demonstrated the identification of mechanical characteristics unique to cancer cells, allowing differentiation between cancerous and normal cells, as well as primary and metastatic cancer cells. This presents a novel complementary method for clinical cancer diagnosis.
Circulating cell-free DNA (ccfDNA), a crucial biomarker for cancer diagnosis and treatment, can reveal modifications to the genotype and phenotype of primary malignancies. Research has shown that the ccfDNA fragmentation index in cancer patients can be found using AFM, suggesting its potential as a biomarker for cancer therapy.
AFM also provides morphological data about the length, width, and height of cells and can be used to examine minute features like receptors, lipid rafts, and different membrane routes. Thanks to AFM's capabilities, researchers can explore the effects of various chemicals on specific cells in a physiological setting.
AFM: Challenges and Innovative Solutions
After almost three decades of development, AFM has become progressively more significant in biomedical research. Nonetheless, challenges remain in realizing its full potential.
Improvements in both temporal resolution and utilization efficiency are essential. Currently, AFM cell measurement is conducted primarily by hand. This includes offline processing of experimental data, manually selecting parameters to create cell force curves, and manually guiding the probes to target cells. As a result, experimental efficiency is significantly constrained.
Multiple clinical examples are necessary to validate the reliability of mechanical property detection for clinical and practical applications. The relative values typical of cancer cells and normal cells must be ascertained, and sample preparation and AFM measurements must be standardized.
Numerous AFM models have been developed recently. Coupled with advancements in complementary techniques, such as integrating AFM with fluorescence and confocal microscopy for correlative microscopy, which compares scans from both devices, AFM is set to address unanswered biological questions in the coming decades.
More from AZoOptics: How Raman and Infrared Spectroscopy Boosts Biomedical Analyses
References and Further Reading
- Xia, F., Youcef-Toumi, K. (2022). Review: Advanced Atomic Force Microscopy Modes for Biomedical Research. Biosensors. doi.org/10.3390/bios12121116
- Deng, X., et al. (2018) Application of atomic force microscopy in cancer research. J Nanobiotechnol. doi.org/10.1186/s12951-018-0428-0
Disclaimer: The views expressed here are those of the author expressed in their private capacity and do not necessarily represent the views of AZoM.com Limited T/A AZoNetwork the owner and operator of this website. This disclaimer forms part of the Terms and conditions of use of this website.