In the modern digital age, where data flows freely and sensitive information is constantly in transit, secure communication has become essential. Traditional encryption methods, while effective, are not immune to the evolving threat landscape. This is where quantum key distribution (QKD) emerges as a revolutionary solution, offering unmatched security for transmitting sensitive data.
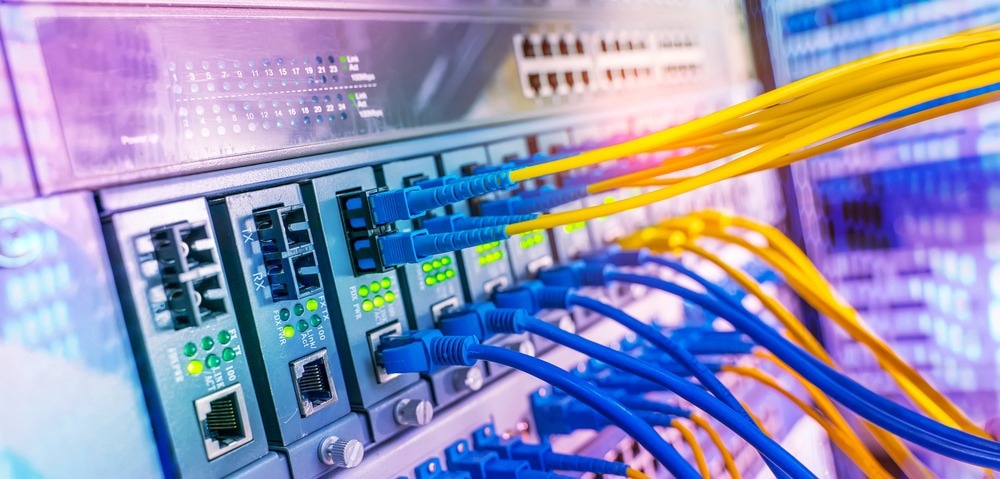
Image Credit: asharkyu/Shutterstock.com
The idea of quantum key distribution (QKD) dates back to Stephen Wiesner's concept of quantum conjugate coding at Columbia University in the 1970s. Charles H. Bennett later built on this idea, introducing the first QKD protocol, BB84, in the 1980s, using nonorthogonal states. Since then, it has matured into one of the most established quantum technologies, commercially available for over 15 years.
How does Quantum Key Distribution Work in Optical Communications?
Quantum key distribution sends numerous photons with random quantum states through fiber optic cables to create a qubit stream. The cryptographic information is encoded into quantum states like photon polarization or phase, and the no-cloning theorem prevents undetectable observation or copying.
Any interception introduces detectable errors, but a well-designed key distribution protocol reveals tampering, ensuring the secure generation of shared random keys over the quantum channel.
In a common QKD implementation, a sender "Alice" encodes photons with one of several quantum states randomly selected from predefined bases—for example, horizontal, vertical, or diagonal polarization. The receiver, "Bob", measures each photon by randomly choosing a measurement basis. Afterward, Alice and Bob communicate their bases publicly without revealing any measurement results.
This allows them to determine which subset of Bob's measurements should perfectly correlate with the states Alice prepared when their bases match. Only keeping these correlated results provides the shared bit values, making up a secret key.
If an adversary "Eve" intercepts and measures some photons mid-transmission, the original quantum state is disturbed based on fundamental restrictions imposed by quantum physics.
When Alice and Bob subsequently reconcile their bases as usual, they will observe an elevated error rate for photons Eve observed, allowing them to detect the potential eavesdropping. The users can then discard compromised key data and continue the protocol until they obtain a secure key through a quantum channel where no tampering is detected.
Advantages of Quantum Key Distribution for Optical Communication
Optical communications infrastructure provides a safe channel for transmitting quantum states for QKD over metro-scale distances. Photonic signals propagating through fiber optic cables experience minimal loss over dozens or even hundreds of kilometers—much further than free-space optical channels. This allows secure key exchange between remote dedicated nodes or sites over an optical backbone network.
Quantum signals comprised of weak photon pulses at one wavelength can share the same optical fiber with traditional high-powered data traffic at other wavelengths with negligible disturbance. This increases capacity and removes the need for separate dedicated dark fibers for key distribution alongside communication links.
The intrinsic sensitivity of quantum states also means infrastructure tampering is inherently detectable. Any attempts to physically split or redirect optical fibers to eavesdrop on quantum channels would impact the detected quantum bit error rates (QBERs), notifying the users. This provides security at both the physical infrastructure and protocol layers when integrated with optical networks.
Together, these advantages enable QKD protocols to unlock unprecedented levels of authenticated confidentiality for messages subsequently encrypted using the generated shared keys and transmitted through the same optical network.
Practical Applications and Case Studies
While fundamental QKD research continues, demonstrations have shown its viability in securing real-world mission-critical optical communication links across industries.
Securing Financial Data Between Remote Sites
Major financial organizations have deployed quantum key distribution to ensure robust encryption protecting sensitive data shared between data centers. For example, Toshiba's QKD technology was implemented on a metro-scale fiber link spanning 32km between Wall Street and New Jersey across the Hudson River. This network connects critical trading infrastructure with back-office operations.
The QKD system leverages wavelength division multiplexing to transmit quantum-secured cryptographic keys alongside traditional high-powered data like transactions, algorithms, and video conferencing streams at different wavelengths. This doubles the capacity compared to separate infrastructure designated only for keys or data.
The installed QKD endpoints maintained an average key generation rate of over 143kbps with minimal errors over sustained real-world operation across the regional financial network. The performance reliably satisfied encryption requirements, demonstrating robust integration with existing optical networking.
Securing Industrial Design Data Sharing
In another industry trial, Toshiba QKD technology was implemented in the UK between the National Composites Centre (NCC) and the Centre for Modelling & Simulation (CFMS). The QKD system distributes thousands of cryptographic keys per second on a standard Openreach fiber.
The QKD link eliminated the manual transport of sensitive design data, securely distributing encryption keys through an optical fiber to enable high-speed encrypted data transfer between sites. In addition, the innovative multiplexing capability enabled the simultaneous transmission of data and quantum keys on the same fiber, eliminating the need for expensive dedicated infrastructure for key distribution.
Toshiba's active stabilization technology ensures the continuous distribution of key material in challenging conditions, eliminating the need for user intervention and recalibration caused by temperature-induced changes in fiber lengths.
Although the initial deployment covers a 7km range, Toshiba's current system can extend to 120km, ensuring ultra-secure data transmission in major metropolitan environments.
Overcoming Challenges in Quantum Key Distribution
While quantum key distribution promises unprecedented security, tangible networks require overcoming complex challenges blending optical engineering and subtle quantum effects.
Most existing systems follow a prepare-and-measure process in which a sender generates a stream of attenuated photons randomly encoded across various bases to distribute a secret key. However, real-world non-ideal optical fibers and detection equipment cause photon loss, dispersion, and noise, reducing secure key rates and distances.
Network designers continue innovating around high-stability interferometers, quantum random number generation, precision timing detectors, and complex software-defined reconciliation protocols to approach theoretical limits.
With ongoing advances and demonstrations, QKD is poised to see increasing real-world adoption in securing fiber optic links across critical infrastructure.
More from AZoOptics: Polarization-Resolved Imaging in Biophotonics
References and Further Reading
NSA. (2023). Quantum Key Distribution (QKD) and Quantum Cryptography (QC). [Online]. Available at: https://www.nsa.gov/Cybersecurity/Quantum-Key-Distribution-QKD-and-Quantum-Cryptography-QC/
Zhang, Q., Xu, F., Chen, Y. A., Peng, C. Z., & Pan, J. W. (2018). Large scale quantum key distribution: challenges and solutions. Optics Express, 26(18), 24260-24273. https://doi.org/10.1364/OE.26.024260
Toshiba. (2023). Achieving secure transfer of sensitive data between remote industrial production facilities. [Online]. Available at: https://www.global.toshiba/ww/products-solutions/security-ict/qkd/cases/case3.html
Toshiba. (2023). Securing the critical link between front office and back office operations of major financial institutions. [Online]. Available at: https://www.global.toshiba/ww/products-solutions/security-ict/qkd/cases/case2.html
Alexander S. Gillis. (2022). Quantum key distribution (QKD). [Online]. Available at: https://www.techtarget.com/searchsecurity/definition/quantum-key-distribution-QKD
Kong, W., Sun, Y., Gao, Y., & Ji, Y. (2023). Coexistence of quantum key distribution and optical communication with amplifiers over multicore fiber. Nanophotonics, 12(11), 1979-1994. https://doi.org/10.1515/nanoph-2023-0047
Guillaume Brochu and Marc-André Laliberté. (2022). Quantum Key Distribution in Optical Communications. [Online]. Available at: https://www.teraxion.com/en/blog/quantum-key-distribution-quantum-communications/
Liu, R., Rozenman, G. G., Kundu, N. K., Chandra, D., & De, D. (2022). Towards the industrialization of quantum key distribution in communication networks: A short survey. IET Quantum Communication, 3(3), 151-163. https://doi.org/10.1049/qtc2.1204
Disclaimer: The views expressed here are those of the author expressed in their private capacity and do not necessarily represent the views of AZoM.com Limited T/A AZoNetwork the owner and operator of this website. This disclaimer forms part of the Terms and conditions of use of this website.