Spectroscopy is one of the primary techniques used in astronomy to understand the Universe. Many breath-taking astronomical discoveries in recent decades resulted from advanced spectroscopic measurements. From the ever-accelerating expansion of the Universe (governed by unknown physics) to the identification and characterization of planets outside our Solar System, these discoveries are just a hint to the unprecedented new opportunities the technological and scientific advances in astronomical spectroscopy promise for the future.
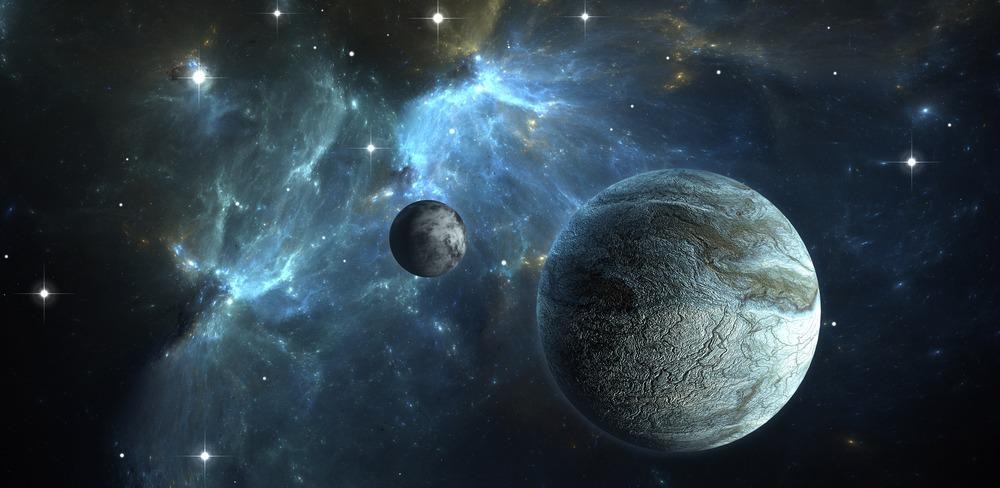
Image Credit: Jurik Peter/Shutterstock.com
Most of the information about astronomical objects outside the Solar System comes through the study of electromagnetic radiation that reaches us. This radiation reveals enormous amounts of information about the celestial objects in the distant cosmos that the astronomers cannot learn any other way.
One of the most versatile tools to extract that information is spectroscopy, which involves dispersing the electromagnetic radiation into its constituent wavelengths (thus obtaining a spectrum). An electromagnetic spectrum contains information about how an object interacts with or emits light, connecting astronomy with fundamental physics at atomic and molecular levels.
Exploring Stellar Spectra
The origin of astronomical spectroscopy can be traced back to the early nineteenth century with the discovery of dark lines in the solar spectrum by W. H. Wollaston in 1802 and J. von Fraunhofer in 1815. The dark lines at discrete wavelengths arise from the absorption of energy by atoms or ions in the solar atmosphere. Shortly after that, fellow scientists observed and analyzed the spectra of other stars and speculated about the stars' chemical composition and structure, thus laying the foundations of modern astrophysics and astronomical spectroscopy.
From the earliest designs to the modern advanced instruments, an astronomical spectrograph consists of four essential components. The light from the telescope is focused onto a slit which permits the selection of the objects to be studied (and helps to remove any stray light). Next, the collimator converts the diverging light beam into a parallel beam. The light reaches a disperser (a prism or a reflection grating), where it splits into its constituent wavelengths. In the end, a detector (whether it will be a photographic film or a modern CCD detector) captures the spectra for visualization and further analysis. Through spectroscopy, astronomers can examine different wavelengths of radiation coming from very distant objects in the Universe, from single stars to massive galaxies, and determine their chemical composition and age, track their motion, and much more.
Clues to the Galactic Evolution
Besides revealing the presence of certain chemical elements, the spectrum of an astronomical object can also inform about the physical properties, such as temperature, density, and magnetic field strength. Careful analysis of many thousands of stellar spectra in the late nineteenth and early twentieth century led to the development of the modern star classification system.
Galactic spectra combine light from billions of stars and all other (emitting or absorbing) matter in the galaxy, such as gas and dust. By examining such data, scientists can follow galactic evolution. The hydrogen spectrum plays a key role in this case, as 90% of the normal matter in the Universe is hydrogen. In a galaxy, only the youngest, hottest stars can excite the surrounding hydrogen gas to emit its characteristic spectrum, thus the strength of the hydrogen emission lines in the spectrum indicates how many young stars are present in the galaxy.
Mapping Motion in the Universe
Spectroscopy can use the Doppler effect (wavelength dependence on the relative motion between the radiation source and the observer) to tell us about the celestial objects' motion through space. If a star or a galaxy is moving away from Earth, scientists will observe a red spectral shift in the object's elemental emission (or absorption) spectrum. Conversely, if the object is moving toward the observer, its spectrum will be blue-shifted. The greater the shift, the greater the object's radial velocity (along the line of sight). Monitoring Doppler shifts in hydrogen atom spectra allowed Edwin Hubble to determine in 1929 that the Universe is uniformly expanding.
The radial velocity measurements are so sensitive that astronomers use them to discover new planetary systems and extrasolar planets. Planets orbiting a star cause an ever so slight wobble in the star's rotation. By observing the stars' spectrum, astronomers can detect wavelength shifts characteristic for the planet-induced wobbling. The degree of the wobble could reveal the size of the planet, while the rate and the variation of wobble carry information about the number of planets and their orbits.
Expanding the Capabilities of Astronomical Spectroscopy
One of the most important advancements in astronomy is the development and use of charge coupling devices (CCDs) as 2D photon detectors for imaging and spectroscopic applications. When used in spectroscopic applications, the CCD detectors significantly increase the sensitivity and throughput of modern telescopes.
The continuing quest for improved productivity and data accuracy in the past two decades led to a technique known as Integral Field Spectroscopy. The technique is a form of hyperspectral imaging that allows signals from multiple astronomical objects to be fed into a spectrograph, which simultaneously generates a spectrum for each object. To provide meaningful data, an IFS array must separate the spatial pixels from each other to produce non-overlapping spectra on the detector. The three current IFS designs originate from three different techniques, using either an array of microlenses, multifiber light guides, or multimirror-based designs.
Many of the large ground-based telescopes are equipped with multiple Integral Field Spectrometers (IFS). The next-generation space telescope, the James Webb Space Telescope (JWST), carries two integral field units operating in the mid-and near-infrared parts of the spectrum. By offering different fields of view and spectral resolutions, these multi-object arrays enable researchers to observe different types of phenomena and solve many astronomical questions simultaneously.
The Challenge of the Exoplanetary Exploration
One of the grand challenges astronomy faces in the future is the discovery and in-depth analysis of Earth-like exoplanets. For the first time, answering questions such as 'How common are the Earth-like worlds in the Universe?' and 'Do any exoplanets show signs of life?' is within our reach.
With more than 4000 exoplanets discovered so far, research groups are rapidly progressing towards detailed spectroscopic observations to characterize the atmospheres of these planets. Space- and ground-based surveys are expected to detect more exoplanets orbiting nearby stars. The forthcoming launch of JWST along with large ground-based facilities is expected to revolutionize exoplanetary spectroscopy.
High-resolution Doppler spectroscopy of planets orbiting close to their stars offers a possibility to detect chemical species in planetary atmospheres. In addition, a combination of high-contrast imaging and high-resolution spectroscopy can be used for the atmospheric characterization of directly imaged planets at large orbital separations. Such observations can provide valuable insights into a wide range of physical processes and chemical compositions in the exoplanetary atmospheres.
New Concepts and Future Prospects for Astronomy
Present-day and near-future major astronomical instrumentation projects, such as the Thirty-Meter Telescope and European Southern Observatory's E-ELT project, are focused on some of the most fundamental research topics, such as explaining the phenomena of dark energy and dark matter, verifying the theory of general relativity, and the formation and evolution of the present Universe (including the formation of stars, planets, and life).
Spectroscopy can contribute significantly to all these topics with some exciting technological developments. Many of the current optical spectrometers designed for multi-object observations require complex mechanisms (movable slits or fibers) that are costly and inconvenient to operate. Significant efforts are devoted to creating simpler and cost-efficient multi-object spectrometers based on micro-electromechanical systems (MEMS). The most advanced MEMS-based micro-shutter array is currently being developed for the JWST's NIRSpec spectrometer.
In the context of optical and X-ray spectroscopy, several types of detectors are under development that can measure the energy (and thus the frequency) of individually observed photons. Finally, scientists plan to harness photonics technology developed and used by the telecommunications industry to create arrayed waveguide gratings (AWGs) that dramatically improve the performance of next-generation astronomical spectrometers.
References and Further Reading
Appenzeller, I. (2012) New Developments and Future Prospects. In Introduction to Astronomical Spectroscopy (Cambridge Observing Handbooks for Research Astronomers, pp. 224-238). Cambridge: Cambridge University Press. Available at https://doi.org/10.1017/CBO9781139059503.011
D. Minardi, et al., (2020) Astrophotonics: astronomy and modern optics. arXiv:2003.12485v2. Available at: https://arxiv.org/abs/2003.12485v2
Madhusudhan, N. (2019) Exoplanetary Atmospheres: Key Insights, Challenges and Prospects. arXiv:1904.03190v1. Available at: https://arxiv.org/abs/1904.03190v1
Y. Cendes (2020) Decadal Survey 2020: The future of astronomy will soon be revealed. [Online] www.astronomy.com Available at: https://astronomy.com/news/2020/08/what-does-the-future-of-astronomy-hold-well-find-out-soon (Accessed on 23 August 2021).
N. Jovanovic, et al., (2020) Status and future developments of integrated photonic spectrographs for astronomy and Earth and planetary sciences", Proc. SPIE 11287, Photonic Instrumentation Engineering VII, 112870K. Available at: https://doi.org/10.1117/12.2546959
Disclaimer: The views expressed here are those of the author expressed in their private capacity and do not necessarily represent the views of AZoM.com Limited T/A AZoNetwork the owner and operator of this website. This disclaimer forms part of the Terms and conditions of use of this website.