How quickly do electrons within a molecule travel? It is extremely quick, about a few attoseconds (1 as = 10–18 seconds or one billionth of a billionth of a second) to move from one atom to the next. One could miss it if they blinked—millions of billions of times. Therefore, measuring such ultrafast processes is a very challenging mission.
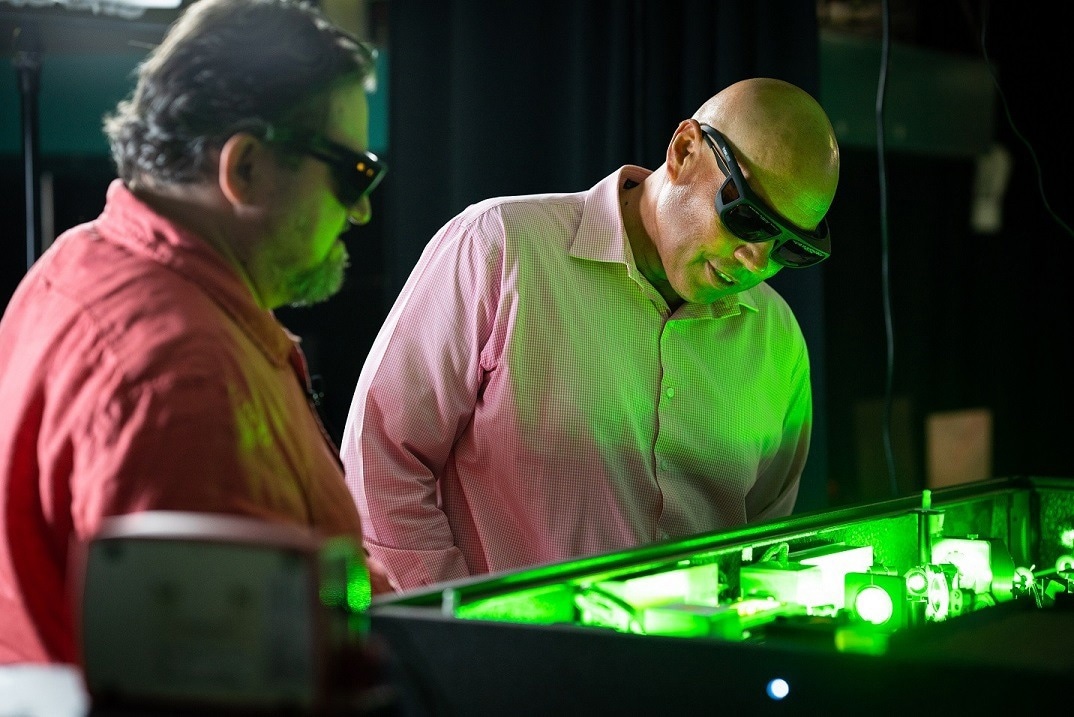
Prof Igor Litvinyuk and Prof Robert Sang. Image Credit: Griffith University
Researchers at the Australian Attosecond Science Facility and the Centre for Quantum Dynamics of Griffith University in Brisbane Australia, guided by Professor Robert Sang and Professor Igor Litvinyuk, have formulated a unique interferometric method that can measure time delays with zeptosecond (a trillionth of a billionth of a second) resolution.
They have applied this method to quantify the time delay between extreme ultraviolet light pulses discharged by two diverse isotopes of hydrogen molecules—H2 and D2—interacting with powerful infrared laser pulses.
This delay was discovered to be less than three attoseconds (one quintillionth of a second long) and is caused by moderately diverse motions of the lighter and heavier nuclei.
Details of this study have been reported in Ultrafast Science, a new Science Partner Journal.
Such unprecedented time resolution is achieved via an interferometric measurement—overlapping the delayed light waves and measuring their combined brightness.
Dr. Mumta Hena Mustary, Study Lead Author, Griffith University
The light waves were generated by molecules when subjected to powerful laser pulses in a process known as high harmonic generation (HHG).
HHG takes place when an electron is eliminated from a molecule by a robust laser field, is quickened by the same field, and then rejoins with the ion discharging the energy in the form of extreme ultraviolet (XUV) radiation.
Both the intensity and phase of that XUV HHG radiation are susceptible to the precise dynamics of the electron wavefunctions incorporated in this process—all diverse atoms and molecules discharge HHG radiation in various ways.
While it is fairly direct to quantify the spectral intensity of HHG—a basic grating spectrometer can accomplish this—quantifying the HHG phase is a much more challenging task. Furthermore, the phase holds the most significant information concerning the timing of several steps in the emission process.
To quantify this phase, it is typical to carry out a so-called interferometric measurement when two imitations of the wave with excellently regulated delay are caused to overlap (or interfere) with each other. They can obstruct destructively or constructively subject to the delay and relative phase variance between them.
Such measurement is carried out by a device known as an interferometer. It is very tough to construct an interferometer for XUV light, particularly to create and preserve a stable, known, and exceptionally tunable delay between two XUV pulses.
The Griffith team solved this issue by exploiting the phenomenon called the Gouy phase—when a phase of a light wave is moved a specific way while going via a focus.
To carry out their experiments, the team used two diverse isotopes of molecular hydrogen—the most basic molecule in nature. The isotopes—light (H2) and heavy (D2) hydrogen—vary only in mass of nuclei—protons in H2 and deuterons in D2. All other aspects including the electronic structure and energies are alike.
Because of their larger mass, the nuclei in D2 travel a little slower than those in H2. Since electronic and nuclear motions in molecules are coupled, nuclear motion impacts the dynamics of the electron wavefunctions during the HHG process resulting in a small phase shift ΔφH2-D2 between the two isotopes.
This phase shift corresponds to a time delay Δt = ΔφH2-D2 /ω where ω is the XUV wave’s frequency. The Griffith researchers quantified this emission time delay for all the harmonics witnessed in the HHG spectrum—it was almost constant and marginally below 3 attoseconds.
To comprehend their outcome, the Griffith scientists were assisted by theorists at Shanghai Jiao Tong University in Shanghai, China, guided by Professor Feng He.
The SJTU researchers applied the most advanced theoretical techniques to expansively model the HHG process in the two isotopes of molecular hydrogen, including all degrees of freedom for electronic and nuclear motion at different levels of approximation.
Their simulation replicated experimental results well, and this agreement between theory and experiment provided the team assurance that the model captured the most vital features of the fundamental physical process, so modifying the model’s parameters and levels of approximation can establish the relative significance of several effects.
While the real dynamics are fairly complex, it was discovered that two-center interference during the electron recombination step is the governing effect.
Because hydrogen is the simplest molecule in nature and it can be modeled theoretically with high accuracy it was used in these proof-of-principle experiments for benchmarking and validation of the method.
Professor Igor Litvinyuk, Study Lead Author, Griffith University
“In the future, this technique can be used to measure ultrafast dynamics of various light-induced processes in atoms and molecules with unprecedented time resolution,” Professor Litvinyuk added.
Journal Reference
Mustary, M.H., et al. (2022) Attosecond Delays of High-Harmonic Emissions from Hydrogen Isotopes Measured by XUV Interferometer. Ultrafast Science. doi.org/10.34133/2022/9834102.