By Ankit SinghReviewed by Susha Cheriyedath, M.Sc.Jul 1 2024
Deoxyribonucleic acid (DNA) origami involves folding long DNA strands into precise shapes and has transformed various fields, including photonics. This technique provides remarkable control at the nanoscale, allowing the construction of complex nanostructures with applications spanning from quantum computing to biomedical imaging. Researchers have leveraged DNA's unique properties to develop complex photonic devices.
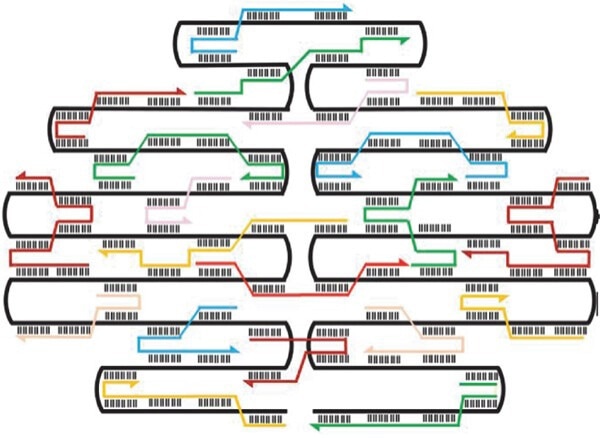
Principles of DNA origami. Image Credit: https://onlinelibrary.wiley.com/doi/10.1155/2021/9112407
This article explores the evolution of DNA origami in photonics, the principles behind its operation, its transformative impact, and current challenges.
How DNA Origami Works?
In DNA origami, a lengthy single-stranded DNA molecule is folded into a specific shape using short, complementary strands called staples. These staples bind to particular sites on the DNA, forcing it to fold into the desired structure. This self-assembly process permits precise nanoscale control, facilitating the positioning of functional components such as fluorescent molecules, nanoparticles, and quantum dots with nanometer precision.1
In photonics, DNA origami functions as a framework for organizing and manipulating light-emitting molecules and other photonic components. By arranging these elements at accurate distances and angles, the technique can enhance light-matter interactions, optimize energy transfer, and enhance the performance of photonic devices. For example, DNA origami can place dye molecules in close proximity, enabling efficient energy transfer and light emission.2
Evolution of DNA Origami in Photonics
The concept of DNA origami was introduced by Paul Rothemund at the California Institute of Technology (Caltech) in 2006. Initially, the technique was predominantly employed in the realm of structural biology to fashion nanoscale shapes and patterns. Over time, researchers recognized its potential in photonics, particularly in positioning and organizing light-emitting molecules and nanostructures with precision.3
Early endeavors concentrated on integrating DNA origami with photonic crystal cavities (PCCs), a type of optical resonator. This integration facilitated the precise placement of fluorescent molecules within PCCs, enhancing their light-emitting properties. The capability to regulate the position and quantity of emitters within a cavity represented a significant advancement, addressing previous reproducibility and precision challenges.2
DNA Origami and Single-Molecule Spectroscopy
In photonics, DNA origami has transformed single-molecule spectroscopy. Scientists have utilized the technique to create plasmonic nanocavities, enhancing the optical fields at the nanoscale. These nanocavities, formed by arranging gold nanoparticles on DNA origami templates, enable the study of single-molecule interactions with unprecedented detail. This approach has been used to detect single DNA bases and amino acid residues, providing valuable insights into molecular behavior and interactions.4
A recent review published in Nanoscale Advances reported the use of DNA origami to produce nanocavities that could amplify the Raman scattering signals from individual molecules. This technique, known as surface-enhanced Raman scattering (SERS), allowed the study of molecular interactions at the single-molecule level, which is crucial for understanding complex biochemical processes.4
Enhancements in Light Emission
DNA origami has also been utilized to enhance light emission in various photonic devices. Researchers have engineered efficient light-emitting systems by strategically placing dye molecules at precise distances on DNA origami structures. These systems can be used in organic light-emitting diodes (OLEDs) and other optoelectronic devices. The precise arrangement of molecules ensures optimal energy transfer and light emission, thereby enhancing the performance of these devices.2
For example, a study published in the Journal of Physical Chemistry C showcased the use of DNA origami to organize dye molecules in a specific pattern, resulting in improved fluorescence properties. This methodology can be applied to create high-efficiency light sources for use in displays, sensors, and other photonic applications.5
Quantum Dot and Fluorophore Integration
Another significant advancement is the integration of quantum dots and organic fluorophores with DNA origami. These nanostructures can be precisely positioned within the origami templates, facilitating the creation of complex photonic systems. Such systems have applications in quantum computing, where controlled light emission and absorption at the nanoscale are crucial. The ability to place single-photon emitters with nanometer precision allows for the development of highly efficient quantum dots and other nanophotonic devices.6
Moreover, scientists are using DNA origami to develop nanoscale arrays of quantum dots. These arrays display improved photoluminescence properties due to the precise placement of quantum dots, minimizing energy loss and maximizing light output. This progression bears significant implications for the advancement of quantum photonic devices, which necessitate precise control over light-matter interactions.6
DNA Origami-based Plasmonics
The field of plasmonics investigates the interaction between the electromagnetic field and free electrons in a metal. DNA origami has been harnessed to fabricate plasmonic nanostructures capable of manipulating light at the nanoscale. By precisely arranging metal nanoparticles on the origami template, researchers have crafted structures that can enhance light-matter interactions, leading to improved sensors and other optical devices.2
Recent studies have shown that DNA origami can be used to create plasmonic waveguides, which can guide light with minimal loss. These waveguides are applicable in optical communication and integrated photonic circuits. Furthermore, plasmonic structures based on DNA origami can be utilized to manufacture highly receptive biosensors for identifying biomolecules at low concentrations.2
A recent study published in Science Advances showcased the use of DNA origami to position gold nanoparticles into bowtie-shaped antennas. These antennas were utilized to produce plasmonic hotspots, which considerably boosted the Raman scattering signals of molecules located within the nanocavities. This method enabled the identification of molecular vibrations with high sensitivity, introducing new opportunities for biochemical sensing and molecular diagnostics.7
Photonic Crystal Structures
Recent research has demonstrated the use of DNA origami to assemble photonic crystal structures with high precision. By arranging DNA-based tetrapods into diamond-like lattices, these structures exhibit outstanding optical properties. The precise management of crystal growth and structure allows for the generation of superior photonic crystals that can be utilized in various optical applications, including waveguides and sensors.2
A recent Science article described the creation of diamond-lattice photonic crystals using DNA origami. These crystals displayed well-defined facets and edges, and their optical properties were significantly enhanced compared to traditional methods. The capacity to produce such high-quality photonic crystals unlocks new opportunities for developing advanced optical devices with superior performance.8
Challenges and Limitations
Despite the significant advancements and potential applications of DNA origami in photonics, several challenges remain. These challenges must be addressed to fully realize the practical applications and commercial viability of DNA origami-based photonic devices.2
The stability of DNA structures under different environmental conditions stands out as a major concern. Enzymes and harsh chemicals can degrade DNA molecules, thus limiting the practical utility of DNA origami-based photonics devices.2
Furthermore, the technique's scalability presents a challenge. While DNA origami enables the precise assembly of nanostructures, producing large quantities for industrial use proves arduous. This difficulty stems from the complexity of the self-assembly process and the demand for precise control over DNA strand folding.2
Additionally, integrating DNA origami with established photonic fabrication methods like lithography requires optimization to ensure compatibility. Furthermore, achieving long-term stability of origami structures in operational environments, such as in vivo applications or industrial settings, is crucial for practical deployment.2
Future Prospects and Conclusion
The future of DNA origami in photonics looks promising as researchers continue to tackle current challenges and expand its applications. Enhancements in the chemical stability of DNA structures and advancements in large-scale production techniques will improve the practical use of DNA origami-based photonic devices.
Furthermore, researchers are investigating innovative ways to combine DNA origami with emerging photonic technologies like integrated photonics and quantum information processing. The precise control provided by this technique could be essential in developing next-generation photonic devices with superior performance and functionality.
In conclusion, DNA origami has emerged as a powerful tool in the field of photonics, enabling the precise assembly of complex nanostructures with diverse applications. From augmenting light emission in optoelectronic devices to enabling single-molecule spectroscopy, this technique has significantly revolutionized photonics. Despite existing challenges, ongoing research and technological progress hold remarkable potential for the future, paving the way for inventive photonic devices that harness the unique capabilities of DNA origami.
References and Further Reading
- Liu, W. et al. (2021). Concepts and Application of DNA Origami and DNA Self-Assembly: A Systematic Review. Appl. Bionics Biomech. DOI: 10.1155/2021/9112407
- Zhao, Z.; Han, Y.; Liu, Y. (2021). DNA origami enabled assembly of nanophotonic structures and their applications. Opt. Mater. Express. 12 (1), 284. DOI: 10.1364/ome.446697
- Zhan, P. et al. (2023). Recent Advances in DNA Origami-Engineered Nanomaterials and Applications. Chem. Rev. DOI: 10.1021/acs.chemrev.3c00028
- Maccaferri, N. et al. (2021). Recent advances in plasmonic nanocavities for single-molecule spectroscopy. Nanoscale Adv. DOI: 10.1039/d0na00715c
- Mathur, D. et al. (2020). Can a DNA Origami Structure Constrain the Position and Orientation of an Attached Dye Molecule? J. Phys. Chem C. DOI: 10.1021/acs.jpcc.0c09258
- Cho, Y. et al. (2022). DNA as grabbers and steerers of quantum emitters. Nanophotonics. DOI: 10.1515/nanoph-2022-0602
- Shen, B. et al. (2018). Plasmonic nanostructures through DNA-assisted lithography. Sci. Adv. DOI: 10.1126/sciadv.aap8978
- Posnjak, G. et al. (2024). Diamond-lattice photonic crystals assembled from DNA origami. Science, 384 (6697), 781–785. DOI: 10.1126/science.adl2733
Disclaimer: The views expressed here are those of the author expressed in their private capacity and do not necessarily represent the views of AZoM.com Limited T/A AZoNetwork the owner and operator of this website. This disclaimer forms part of the Terms and conditions of use of this website.