Time-resolved spectroscopy (TRS) is a powerful analytical technique that has found its way into various scientific disciplines. Among these, biology has emerged as a promising domain where time-resolved spectroscopy offers valuable insights into complex biological processes. This article discusses time-resolved spectroscopy in biology, providing a comprehensive understanding of its significance, recent trends, technical details, and recent studies.
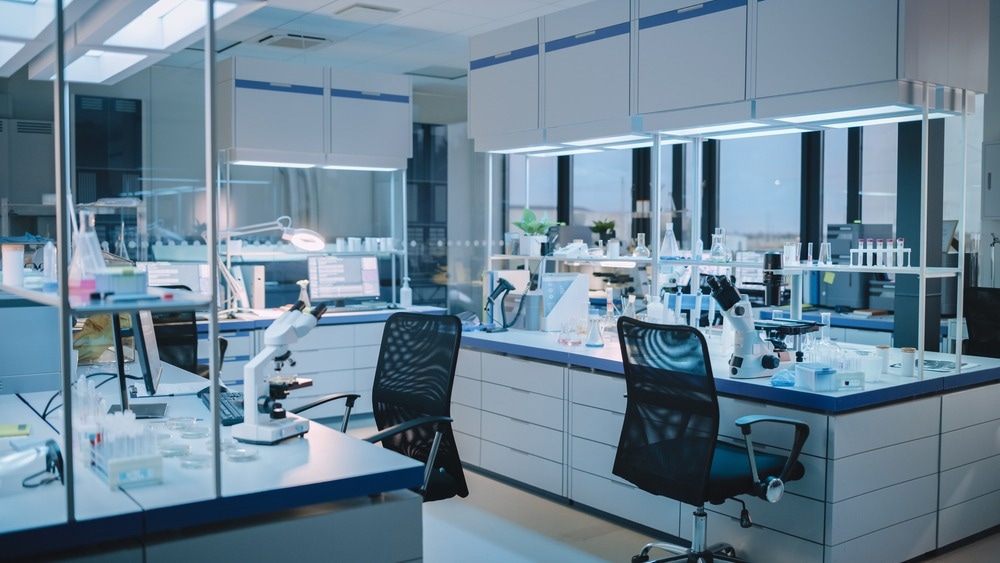
Image Credit: Gorodenkoff/Shutterstock.com
Advantages of Time-Resolved Spectroscopy
In biology, many vital processes happen in milliseconds, microseconds, or even femtoseconds. Time-resolved spectroscopy can capture these fleeting moments with incredible precision. For instance, it can reveal the kinetics of enzymatic reactions, electron transfer in photosynthesis, or protein folding - all in real-time.
Moreover, Time-resolved spectroscopy is not limited to monitoring kinetics alone but also unveils structural changes within biomolecules. This is particularly important in studying protein conformational changes, which play a crucial role in various diseases. Time-resolved spectroscopy can also detect changes in pH, ion concentration, and temperature, offering insights into how these factors affect biochemical reactions. Another crucial advantage of Time-resolved spectroscopy is in drug development and disease studies since it helps understand how drugs interact with their target molecules in real-time. For instance, it has applications in cancer research, where it can monitor the dynamics of cell proliferation and migration.
Recent Trends in Time-Resolved Spectroscopy in Biology
Recent developments have seen the integration of various spectroscopic techniques with Time-resolved spectroscopy, allowing for a more comprehensive understanding of biological processes. Combining Time-resolved spectroscopy with other methods like NMR, X-ray crystallography, and electron microscopy provides a multi-dimensional view of complex biological systems.
Similarly, single-molecule Time-resolved spectroscopy has gained momentum, enabling researchers to monitor individual molecules and observe their behavior in great detail, especially in studying biomolecular interactions and conformational changes.
Another emerging trend is combining Super-resolution microscopy with Time-resolved spectroscopy to achieve spatial resolutions far beyond the diffraction limit. This has opened new horizons in studying subcellular structures and cellular processes, allowing researchers to visualize the previously unseen.
Recent trends are leaning towards in vivo applications of Time-resolved spectroscopy as well. This involves the non-invasive monitoring of biological processes within living organisms, making it a potential game-changer in fields like neuroscience, pharmacology, and medical diagnostics.
How Time-Resolved Spectroscopy in Biology Works
Time-resolved spectroscopy relies on the interaction between matter and electromagnetic radiation. Pluses of light as brief as femtoseconds are generated in the form of ultra-short laser pulses, allowing the capture of ultrafast events. A laser pulse is directed at the biological sample, which interacts with the sample, inducing changes that affect the properties of the probing light, such as its wavelength, polarization, or intensity.
A second pulse of light, often referred to as the probe pulse, is used to measure the changes in the properties of the initial pulse. The time delay between the pump (excitation) and probe pulses is precisely controlled. Hence, the data collected is analyzed to reconstruct the temporal evolution of the sample's properties, providing insights into the dynamic processes taking place in the biological system.
Recent Research in Time-Resolved Spectroscopy
In a 2021 study, researchers explored the applications of Time-resolved spectroscopy in the field of biology, focusing on optogenetic channels, specifically channelrhodopsins (ChRs) and anion channelrhodopsins (ACRs). They investigated the photocycle of the natural ACR, Guillardia theta Anion Channelrhodopsin-1(GtACR1), and compared it with the well-studied ChR (Chlamydomonas reinhardtii Channelrhodopsin-2), CrChR2. These are specific types of proteins or channelrhodopsins. They conducted time-resolved UV/VIS and FTIR spectroscopy to monitor the dynamic molecular processes during the photocycle of GtACR1.
The study revealed the absence of a light-adapted state in GtACR1, distinguishing it from CrChR2. GtACR1 demonstrated higher peak currents and reduced inactivation, which are attributed to its preexisting tunnel and the absence of a syn-photocycle. The research provided insights into the molecular mechanisms of GtACR1's channel gating and its differences from CrChR2, enhancing the understanding of optogenetic tools' design and applications in biology.
Future Prospects of Time-Resolved Spectroscopy
The future prospects of Time-resolved spectroscopy in biology are exceptionally promising. Time-resolved spectroscopy is poised to revolutionize the study of complex biological processes, offering invaluable insights and applications. As technology advances and research in this field evolves, several exciting developments can be anticipated, including enhanced multimodal integration, as Time-resolved spectroscopy combines with other advanced spectroscopic techniques like NMR, X-ray crystallography, and electron microscopy. This integration will provide a more comprehensive understanding of complex biological systems.
Similarly, the shift towards in vivo applications involving non-invasive monitoring of biological processes within living organisms holds immense potential in fields such as neuroscience, pharmacology, and medical diagnostics. These advancements will collectively shape and enhance our understanding of biological processes, offering new avenues for research and applications in biology.
More from AZoOptics: Raman Spectroscopy for Label-Free Chemical Analysis
References and Further Reading
Dreier, M. A., Althoff, P., Norahan, M. J., Tennigkeit, S. A., El-Mashtoly, S. F., Lübben, M., ... & Gerwert, K. (2021). Time-resolved spectroscopic and electrophysiological data reveal insights in the gating mechanism of anion channelrhodopsin. Communications Biology. https://doi.org/10.1038/s42003-021-02101-5
Schmitt, M., Dietzek, B., Hermann, G., & Popp, J. (2007). Femtosecond time‐resolved spectroscopy on biological photoreceptor chromophores. Laser & Photonics Reviews. https://doi.org/10.1002/lpor.200710005
Gupta, B. K., Rathee, V., Narayanan, T. N., Thanikaivelan, P., Saha, A., Govind, ... & Ajayan, P. M. (2011). Probing a Bifunctional Luminomagnetic Nanophosphor for Biological Applications: a Photoluminescence and Time‐Resolved Spectroscopic Study. https://doi.org/10.1002/smll.201100441
Masuch, R., & Moss, D. A. (2003). Stopped flow apparatus for time-resolved Fourier transform infrared difference spectroscopy of biological macromolecules in 1H2O. Applied spectroscopy. https://doi.org/10.1366/000370203322554581
Disclaimer: The views expressed here are those of the author expressed in their private capacity and do not necessarily represent the views of AZoM.com Limited T/A AZoNetwork the owner and operator of this website. This disclaimer forms part of the Terms and conditions of use of this website.