A photonic crystal is primarily an artificially synthesized structure possessing a refractive index modulated with a period that is comparable to the wavelength of light in the material. These crystals are fabricated using state-of-the-art (SOTA) semiconductor microfabrication technologies. This article discusses photonic crystals and their role in controlling the propagation of light.
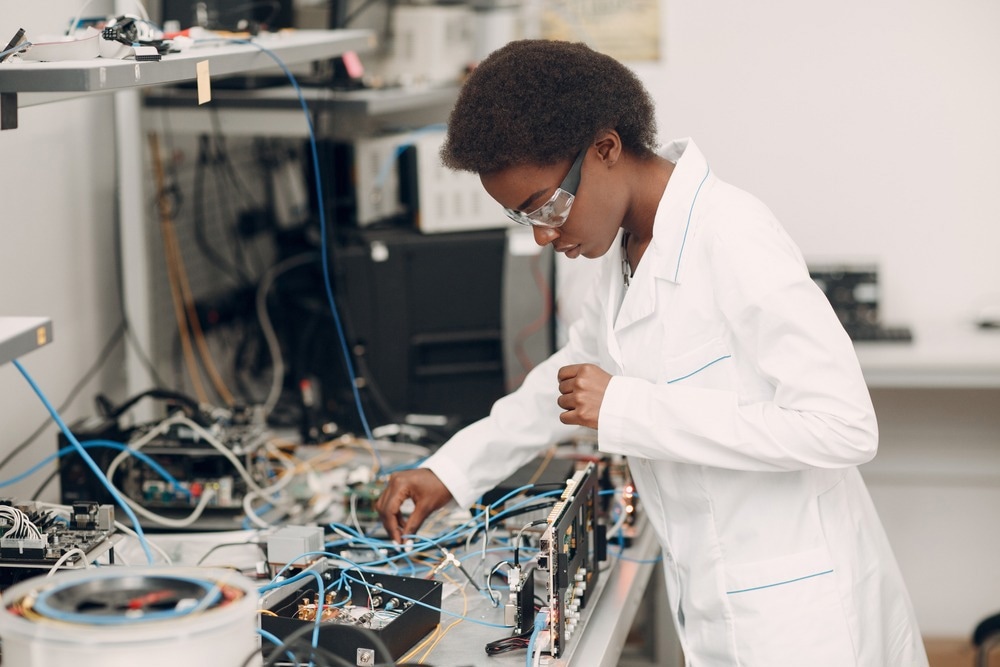
Image Credit: Max4e Photo/Shutterstock.com
Importance of Photonic Crystals
In photonic crystals, periodicity similar to the optical wavelength is introduced artificially to ensure the formation of a photonic band structure. The dispersion of light within a photonic crystal demonstrates the formation of a photonic band structure. The presence of a photonic bandgap is one of the critical aspects of band formation.
Specifically, the photonic crystal must behave as a photonic insulator in the gap wavelength region where the propagation of light is inhibited without any absorption loss. Additionally, the light propagation can be manipulated significantly outside the bandgap.
Controlling Light Propagation Using Photonic Crystals
The photonic crystal structure offers a periodic variation in refractive index to propagating photons. The periodicity can range from centimeters to tens of nanometers, depending on the application. Structural periodicity engineered intelligently can inhibit the propagation of electromagnetic radiation within a material in one, two, or all three dimensions.
Photonic crystals can effectively reflect specific wavelength bands due to the photonic bandgap created by their periodic structure. The nature of the wavelength band reflected by the photonic crystal depends on three variables, including crystal design, the refractive index difference of constituent materials, and the relative volume occupied by the constituent materials in the crystal.
Crystal design is crucial as different directions through a photonic crystal correspond to different periodic spacings. The refractive index difference and relative volumes of constituent materials influence the nature of the non-propagating/standing waves within the crystal.
Bragg diffraction
Bragg diffraction occurs in three dimensions, and a complete three-dimensional (3D) photonic bandgap is realized for the same wavelength band when the correct combination of all three variables is selected for a photonic crystal. A 3D photonic bandgap prevents the propagation of a specific electromagnetic radiation band through the crystal in any direction.
The phenomenon can be exploited for different applications, such as designing and incorporating a nanoscale path in a device to guide a light beam/pulse from one place to another place mediated by Bragg diffraction.
Strongly modulated photonic crystals, such as two-dimensional (2D) hexagonal pillar gallium arsenide (GaAs) crystals, demonstrate negative refraction near the photonic bandgaps, where the light beam is refracted in the opposite direction.
Additionally, the negative refraction produces different unconventional light propagation phenomena, such as the convergence of a point source generated light in a negative-index photonic crystal, with the flat negative-index material surface acting as a lens.
This flat lens is different from a conventional lens as it does not have a principal axis or a specific focal length. Thus, the negative-index lens produces a real mirror-inverted image in the negative-index material.
Strong light confinement can be achieved in 2D photonic bandgap materials precisely fabricated using complementary metal-oxide semiconductor (CMOS) fabrication technologies. Although perfect bandgap confinement cannot be achieved in 2D systems, several steps can be implemented to optimize the 2D bandgap-produced confinement.
For instance, the ultrahigh-quality factor (Q) optical cavity in the 2D photonic crystal is based on a theoretically loss-free line-defect waveguide. The termination of the waveguide can deform the original loss-free mode profile, leading to significant out-of-plane radiation loss.
To address this issue, the position of a specific number of air holes can be slightly shifted by a few nanometers in place of terminating the waveguide to prevent the alteration of the original loss-free mode profile and create a strongly confined cavity mode.
Photonic crystals can substantially alter the group velocity by the photonic band formation, leading to significant light speed reduction. A resonator can also be used to realize dispersion-managed slow-light modes.
A resonator induces a group delay of twice the cavity’s photon lifetime at the resonance frequency. Additionally, no group velocity dispersion occurs at the resonance frequency. Thus, high- and small-Q cavities can generate dispersion-free slow light. For instance, ultrahigh-Q nanocavities in photonic crystals can substantially reduce the light speed due to the small size of the cavity and ultrahigh-Q value.
Recent Studies
The spatially variant photonic crystals (SVPCs) have demonstrated the ability to control the direction and propagation of light in the near-infrared spectrum. In a study recently published in the journal Scientific Reports, researchers designed spatially-varied, unique unit-cell structures to develop photonic crystals that maintain direction and self-collimation of light for desired beam-tuning applications.
Researchers used the finite-difference time-domain technique to predict the beam-bending and self-collimation capabilities of the synthesized SVPCs. The SVPC designs and simulation results were verified by laboratory testing.
Results displayed that the 2D SVPCs can attain self-collimation and direct light through sharp bends. Moreover, the quality and simplicity of the SVPC designs allow their implementation in modern devices. These photonic crystals can be used as a novel solution to optical systems for data transfer, multiplexing, and optical computing.
More from AZoOptics: How is Electron Microscopy Used for Fiber and Textile Analysis?
References and Further Reading
Volk, A., Rai, A., Agha, I., Payne, T. E., Touma, J. E., Gnawali, R. (2022). Development of spatially variant photonic crystals to control light in the near-infrared spectrum. Scientific Reports, 12(1), 1-11. https://doi.org/10.1038/s41598-022-20252-1
Vukusic, P. (2006). Manipulating the flow of light with photonic crystals. Physics Today, 59(10), 82–83. https://doi.org/10.1063/1.2387101
Notomi, M. (2009). Manipulating Light by Photonic Crystals [Online] Available at https://www.ntt-review.jp/archive/ntttechnical.php?contents=ntr200909rp1.html (Accessed on 09 August 2023)
Disclaimer: The views expressed here are those of the author expressed in their private capacity and do not necessarily represent the views of AZoM.com Limited T/A AZoNetwork the owner and operator of this website. This disclaimer forms part of the Terms and conditions of use of this website.