Dynamically tunable optical filters have gained considerable attention as alternatives to static filters. The spectral transmittance or reflectance functions of dynamic filters can be modified to give a dual-purpose functionality to an imaging system. However, tunable filters are highly challenging to fabricate. This article looks at Heenkenda, R. et al.'s paper published in Optics Express.
Materials with a refractive index that can be electronically modified are uncommon. Moreover, it is much more uncommon to find those that are compatible with standard deposition methods, can be combined into typical multilayer optical stacks, and can be used in an end application.
This study describes an innovative tunable optical filter designed for smart IR night vision imaging systems. For this purpose, the chalcogenide class of materials, including Ge, Sb, and Te (generally abbreviated as GST) are considered.
GSST (which stands for Ge, Sb, Se, and Te) is a novel phase change material (PCM) that sustains a similar phase transition, yet at a higher transition temperature of about 300 °C rather than 150 °C. GSST was selected as the active optical material in this study.
The filter design proposed in this study is a Metal-Insulator-Metal (MIM) cavity that can be fabricated easily in the form of stratified layers without using any complicated patterning processes. This study introduces a refined theory not just for developing MIM but also for integrating PCMs that can easily adapt to other configurations, wavelengths, and applications.
Methodology
For the application proposed in this study, silicon-based cameras essentially covering visible and near-infrared (NIR) wavelengths of up to 1 μm were considered. The aim of this study is to facilitate night vision while maintaining appropriate daytime color imaging.
Traditional color cameras based on a short-pass filter are not compatible with this dual-band operation. A more potential solution to overcome this issue is to use a dynamically tunable optical filter with the ability to attenuate or pass near-IR wavelengths in the absence of any moving parts.
This article discusses the design, fabrication, and imaging performance of an 850-nm switchable optical notch filter based on a phase change material (i.e., GSST). This filter was mainly designed to pass light with a wavelength close to 850 nm in its crystalline state while attenuating it in its amorphous state.
The new tunable GSST filter has been designed based on the MIM resonant optical cavity. It is feasible to systematically design MIM cavities with the help of the effective reflectance index technique.
A solved contour plot of MIM cavity designed using Ag(M1)/TiO2(H)/Ag(M2) at a wavelength of 850 nm is shown in Figure 1.
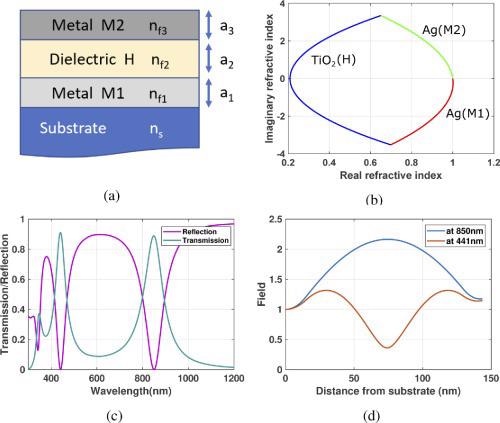
Figure 1. (a) MIM single-cavity structure with thicknesses a1, a2, and a3 with refractive indices nf1, nf2, and nf3, (b) Solved contour plot for the MIM cavity using effective reflectance index method, (c) Reflection/transmission plot using TMM for the structures in (a) and (d) Field intensity plot for the cavity. Image Credit: Heenkenda, et al., 2021
A redesigned contour plot for 850 nm using a thin layer of GSST is illustrated in Figure 2(a). Figure 2(b) shows that while it exhibits a fundamental resonance at 850 nm, a second-order resonance is exhibited at 419 nm.
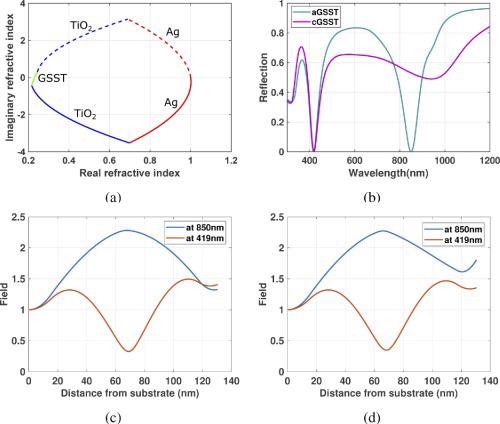
Figure 2. (a) Solved contour of the structure after adding an a-GSST layer at the center of the cavity; (b) Reflection spectrum in the a-GSST and c-GSST states; Field plot for the cavity in the (c) a-GSST state, and (d) c-GSST state. Image Credit: Heenkenda, et al., 2021
The structure in Figure 2 was used as a baseline to create a tunable filter for imaging applications at both day and night time and it was improved further for a silicon substrate exhibiting resonances at 400 and 850 nm. An oblique incidence is more suitable for a reflective configuration, so the filter was designed for an incident angle of 45°. Figure 3 illustrates the final structure of the filter.
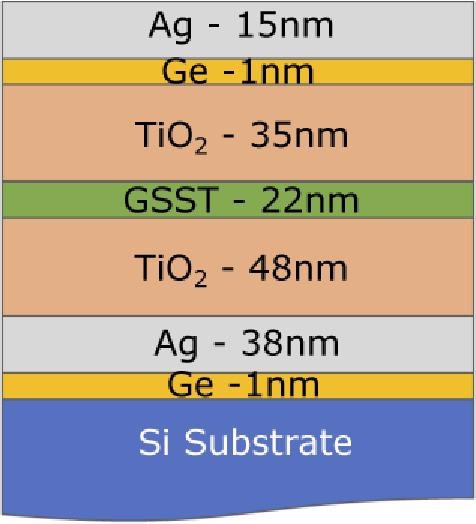
Figure 3. Final layer structure of the filter with additional Ge layers to improve silver wetting designed for 45° incidence. Image Credit: Heenkenda, et al., 2021
Some changes in thickness are unavoidable as part of the actual device fabrication. Hence, a simple configuration including a spectrometer, a white light source, and a base mirror was used to measure the fabricated filter’s reflection spectrum at various incident angles to select the ideal angle (20°) for the desired application. This has been illustrated in Figures 4(a) and 4(b).
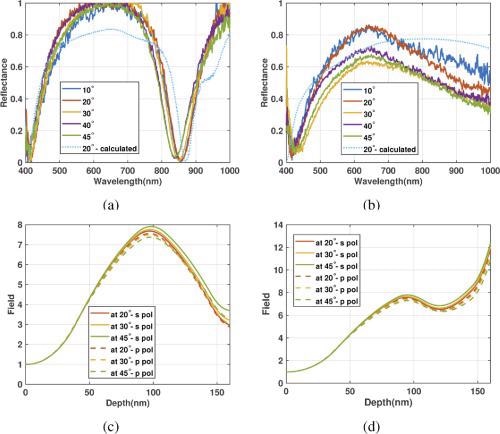
Figure 4. Measured spectra of (a) a-GSST and (b) c-GSST filter samples at different incident angles, including the calculated values for 20° incidence of unpolarized light. Image Credit: Heenkenda, et al., 2021
Results
Figure 5(a) shows the imaging configuration used in this study. As illustrated in Figure 6(a), a dual-bandpass filter with a spectral transmission was used. Figure 6(b) shows that the combination of the dual-bandpass filter and a-GSST filters offer an overall effect similar to a traditional short-pass filter found in color cameras. Thanks to this configuration, the silicon detector is sensitive to an external NIR illumination source.
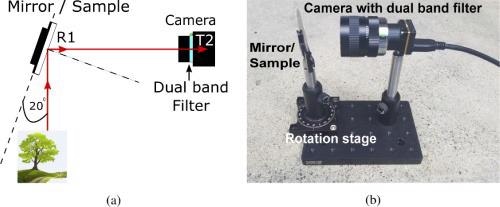
Figure 5. Prototype of the image demonstration setup. R1 is the reflection from the sample/mirror and T2 is the transmission through the dual-band filter (D850). Image Credit: Heenkenda, et al., 2021
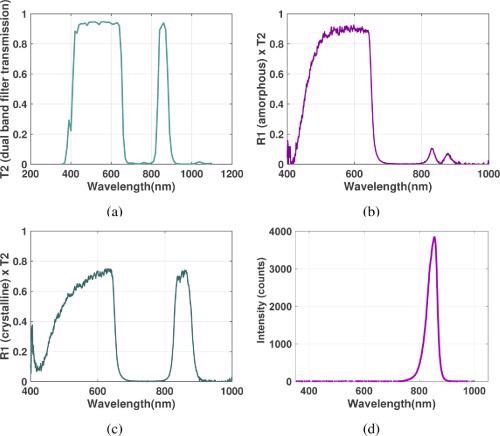
Figure 6. Measured spectra of filters used in the imaging configuration illustrated in Figure 5. (a) Transmission spectra (T2) of Midwest Optical System D850 static filter. (b) Fraction of photons that reach the detector after reflecting from the GSST filter (amorphous state, or a-GSST) and passing through D850 static filter. (c) Fraction of photons that reach the detector after reflecting from the GSST (crystalline state, or c-GSST) filter and passing through D850 static filter. (d) Emission spectrum of the 850 nm LED used in this experiment. All reflection and transmission values shown are in absolute units. Image Credit: Heenkenda, et al., 2021
Figure 7 illustrates some images captured during the daytime and nighttime to evaluate the filtered imaging performance.
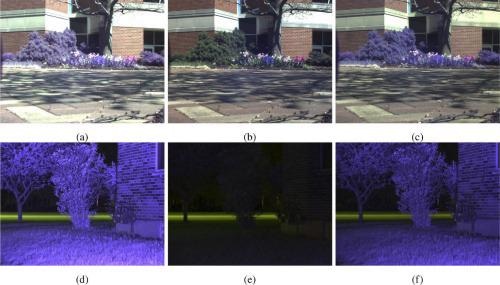
Figure 7. Images captured by prototype system in Figure 5. Daytime color images under solar illumination taken using (a) Ag control mirror, (b) a-GSST mirror, and (c) c-GSST mirror. Nighttime images with 850 nm LED illumination taken using (d) Ag control mirror, (e) a-GSST mirror, and (f) c-GSST mirror. Due to the blocked NIR wavelengths, leaves in (b) appear green but the image in (e) is dark. Image Credit: Heenkenda, et al., 2021
The NIR wavelength on the leaves exhibit high reflectivity, which together with strong solar NIR illumination renders a strong violet hue in the control (see Figure 7(a)) and c-GSST (see Figure 7(c)) images. On the other hand, the newly conceived a-GSST filter passes only visible light, which makes the color of leaves in Figure 7(b) green as predicted usually.
The a-GSST and dual-bandpass filter combination totally rejects the NIR illumination source, resulting in the dark image shown in Figure 7(e). By contrast, the camera fitted with c-GSST and the dual-bandpass filter successfully yielded images of much higher brightness (see Figure 7(f)).
Conclusion
This study demonstrated an innovative optical filter that functions in the reflection mode with a tunable notch at a wavelength of 850 nm. It works based on the GSST phase change material. Heenkenda, R. et al. designed a prototype imaging system for daytime (color image) and nighttime (NIR illumination) imaging.
A CMOS camera and visible + 850-nm dual-bandpass filter combination helped show that in the a-GSST state, color imaging is precise during the daytime, while in the c-GSST state, nighttime NIR imaging is viable with active 850-nm illumination.
Journal Reference:
Heenkenda, R., Hirakawa, K., Sarangan, A. (2021) Tunable optical filter using phase change materials for smart IR night vision applications. Optics Express, 29(21), pp. 33795–33803. Available online: https://www.osapublishing.org/oe/fulltext.cfm?uri=oe-29-21-33795&id=460096.
References and Further Reading
- Kolomiets, B T (1964) Vitreous semiconductors (I). Physica Status Solidi B, 7(2), pp. 359–372. doi.org/10.1002/pssb.19640070202.
- Yamada, N (1996) Erasable phase-change optical materials. MRS Bulletin, 21(9), pp. 48–50. doi.org/10.1557/S0883769400036368.
- Williams, C., et al. (2020) Tunable mid-wave infrared Fabry-Perot bandpass filters using phase-change GeSbTe. Optics Express, 28(7), pp. 10583–10594. doi.org/10.1364/OE.390538.
- Julian, M. N., et al. (2020) Reversible optical tuning of GeSbTe phase-change metasurface spectral filters for mid-wave infrared imaging. Optica, 7(7), pp. 746–754. doi.org/10.1364/OPTICA.392878.
- Mou, N., et al. (2020) Large-scale, low-cost, broadband and tunable perfect optical absorber based on phase-change material. Nanoscale, 12(9), pp. 5374–5379. doi.org/10.1039/C9NR07602F.
- Zhang, H., et al. (2019) Nonvolatile waveguide transmission tuning with electrically-driven ultra-small GST phase-change material. Science Bulletin, 64(11), pp. 782–789. doi.org/10.1016/j.scib.2019.04.035.
- Chu, C. H., et al. (2016) Active dielectric metasurface based on phase-change medium. Laser & Photonics Reviews, 10(6), pp. 986–994. doi.org/10.1002/lpor.201600106.
- He, Q., et al. (2020) Dynamically tunable transmissive color filters using ultra-thin phase change materials. Optics Express, 28(26), pp. 39841–39849. doi.org/10.1364/OE.411874.
- Zhang, Y., et al. (2019) Broadband transparent optical phase change materials for high-performance nonvolatile photonics. Nature Communications, 10(1), p. 4279. doi.org/10.1038/s41467-019-12196-4.
- Zhang, Y., et al. (2021) Electrically reconfigurable non-volatile metasurface using low-loss optical phase-change material. Nature Nanotechnology, 16, pp. 661–666. doi.org/10.1038/s41565-021-00881-9.
- Qu, Y., et al. (2017) Dynamic thermal emission control based on ultrathin plasmonic metamaterials including phase-changing material GST. Laser & Photonics Reviews, 11(5), p. 1700091. doi.org/10.1002/lpor.201700091.
- Carrillo, S. G.-C., et al. (2019) A nonvolatile phase-change metamaterial color display. Advanced Optical Materials, 7(18), p. 1801782. doi.org/10.1002/adom.201801782.
- Sarangan, A (2018) Design of metal-dielectric resonant-cavity thin-film structures using the effective reflectance index method. Journal of the Optical Society of America B, 35(9), pp. 2294–2301. doi.org/10.1364/JOSAB.35.002294.
- Sarangan, A (2020) Optical Thin Film Design (1st ed.). CRC Press.
- Chen, W., et al. (2010) Ultra-thin ultra-smooth and low-loss silver films on a germanium wetting layer. Optics Express, 18(5), pp. 5124–5134. doi.org/10.1364/OE.18.005124.
- Ni, C., et al. (2014) Effects of different wetting layers on the growth of smooth ultra-thin silver thin films. Proceedings of SPIE 9170, Nanoengineering: Fabrication, Properties, Optics, and Devices XI, 91700L. doi.org/10.1117/12.2061256.
- Yang, R., et al. (2020) Planar ultrathin omni-directional perfect absorber utilizing amorphous silicon for photovoltaics. Optical Materials Express, 10(2), pp. 532–539. doi.org/10.1364/OME.382506.