Transistors are semiconductor devices that have revolutionized the electronics industry. These small, often innocuous devices are capable of acting as rapid switches for controlling information flow as well as amplification and modulation steps for signals. Transistors are at the heart of computing, where they act as a mechanism for the storage and movement of data.
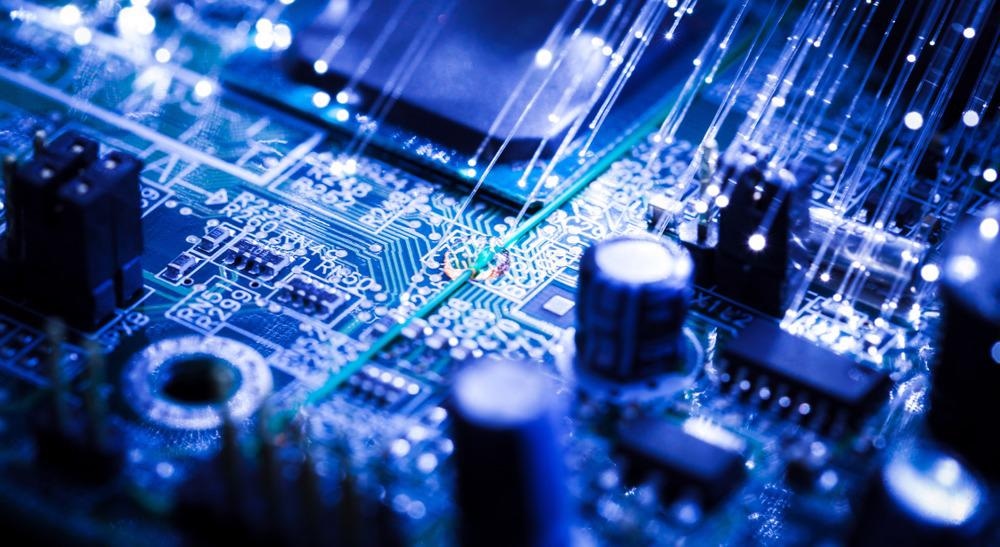
Image Credit: AerialVision_it/Shutterstock.com
The miniaturization of transistors has played an important role in the acceleration of computing power which occupies the same volume of space. In 1965, Gordon Moore proposed a law that the number of transistors on a given chip area would double each year, leading to the rapid acceleration in computing power we have seen in recent decades.
However, Moore’s Law is beginning to reach its limits as transitions are reaching the nanometer scale. Such small transistors struggle to contain the electrical currents that flow through them, leading to heat generation and leakage.
Reaching the desired density of transistors on a chip only serves to amplify this problem as the thermal load becomes so uncontrollable the only possibilities are to throttle power to the chip or use only a subset of the available transistors. Fabricating nanoscale devices is also very expensive, and so the pursuit of this continual miniaturization to achieve greater computing outputs has become less appealing.
While many researchers are working on new fabrication and semiconductor design approaches to deal with this challenge,1 completely new architecture has been developed. One of these is the use of optical transistors.
Optical Information
Light has already become the medium of choice in much of the telecommunications industry. Rather than transmitting information as an electrical signal through thick copper bundles, data is encoded in sequences of light pulses that are transmitted through kilometers of fiber optics.
Light has several advantages over electricity for information transfer. It travels at much greater speeds so faster communications are possible, and much greater bandwidth transmission can be achieved. This means more information can be encoded and sent.
There are fewer issues with thermal load in fiber optics and, for transmission of information through conductive wires, these thermal losses pose another issue – information loss. Fiber optics have greater transmission and so require fewer signal boosts over an equivalent length network.
Optical Transistors
Optical transistors are the light-based equivalent of the electrical transistor but can be used to manipulate the properties of light passing through. However, while the concept of a complete optical logic gate that can achieve all the same functionality as an electrical transistor has proved highly appealing, there are very few optical devices that have achieved this.2
The other challenge such devices face is that a single transistor will not be sufficient. To truly supersede electrical transistors, it must be possible to create chips with numerous optical transistors integrated.
Finding new materials that might offer the level of optical control required for such a device has been a great challenge but recently, a team of researchers from both Skoltech and IBM has created an incredibly efficient optical switch that may pave the way to new optical transistors.
The team demonstrated that its new switching device was capable of operating 100-1000 times faster than current commercial transistors and was incredibly energy efficient. The device could be switched using just a few photons meaning it could be operated at ambient temperatures with no huge thermal load to deal with. Trying to cope with the issue and energy demand of cooling huge server rooms for data transfer due to current transistor designs is a significant challenge.
Switching Behavior
As well as the rapid switching, the team showed that its new optical transistor design could amplify a laser beam by up to 23,000 times. The trick to achieving all these functionalities for such a low energy cost was in the molecular design of the transistor.
The team used a polymer in a specially designed microcavity to exploit a phenomenon known as strong-field coupling. When a molecule is placed inside an optical cavity and the size of the cavity is tuned to match the frequencies of the optical absorption of the molecule, there can be a strong interaction between the modes of the cavity and the molecular species leading to the formation of polaritonic states.
The molecular species also has its own vibrational modes or excitons, and these states can be perturbed by the interactions between them and the states in the cavity. The team used extremely weak laser pulses to stimulate these excitonic-polaritonic states and induce rapid, sub-picosecond switching in the system.
The Future of Optical Transistors
There are still many challenges to overcome with the fabrication and creation of such microcavities and a need to develop our understanding of the mechanisms involved in these strong field interactions. However, given the properties of the excitonic-polaritonic states can be tuned with the cavity size, there are many exciting possibilities of the use of this technology for future optical transitions and the field has undergone many rapid developments in the last few years.
References and Further Reading
- Lambrechts, W., Sinha, S., Abdallah, J. A., & Prinsloo, J. (2018). Extending Moore’s Law through Advanced Semiconductor Design and Processing Techniques. CRC Press. https://books.google.co.uk/books?id=NEkPEAAAQBAJ
- Miller, D. A. B. (2010). Are optical transistors the logical next step? Nature Photonics, 4(1), 3–5. https://doi.org/10.1038/nphoton.2009.240
- Zasedatelev, A. V., Baranikov, A. V., Sannikov, D., Urbonas, D., Scafirimuto, F., Shishkov, V. Y., Andrianov, E. S., Lozovik, Y. E., Scherf, U., Stöferle, T., Mahrt, R. F., & Lagoudakis, P. G. (2021). Single-photon nonlinearity at room temperature. Nature, 597(7877), 493–497. https://doi.org/10.1038/s41586-021-03866-9
Disclaimer: The views expressed here are those of the author expressed in their private capacity and do not necessarily represent the views of AZoM.com Limited T/A AZoNetwork the owner and operator of this website. This disclaimer forms part of the Terms and conditions of use of this website.