An international team of researchers from Australia and Germany recently developed a microscopy technique that employs quantum-correlated illumination to image nano-scale biological structures at an unparalleled resolution without any light-induced damage.
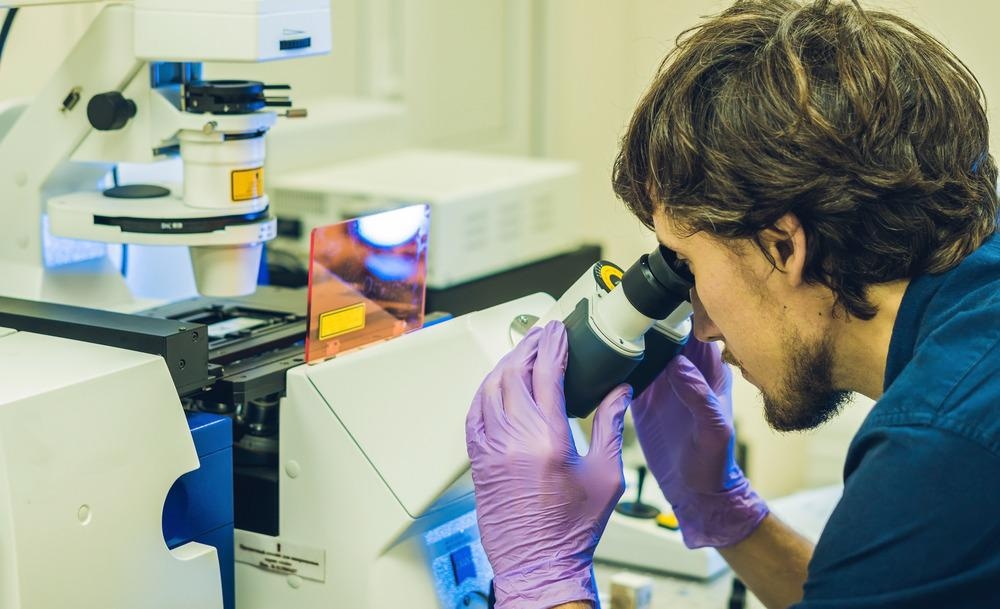
Image Credit: Elizaveta Galitckaia/Shutterstock.com
State-of-the-art optical microscopes use high-powered lasers to examine biological specimens in greater detail. Modern super-resolution microscopy techniques allow visualization of subcellular organization with unprecedented detail, but also confront scientists with the challenge of preventing photodamage in fragile live-cell specimens.
Fluorescence (absorption and subsequent emission of light by organic and inorganic specimens) is the mainstay of optical microscopy that underpins some of the most spectacular advances in super-resolution imaging of biological systems over the past two decades.
Super-Resolution Breakthrough
Fluorescence microscopy emerged as a universal tool particularly in life sciences because of its unique ability to map a wide variety of target biomolecules as well as the physical structure of complex systems. In the majority of fluorescence microscopy applications, such as confocal laser scanning microscopy (CLSM) and total internal reflection fluorescence (TIRF) microscopy, a laser is used as an illumination source because of its unique combination of ultra-high brightness and spectral monochromaticity.
The development of super-resolution techniques such as photoactivated localization microscopy (PALM) and stimulated emission-depletion (STED), awarded with the 2014 Nobel Prize in Chemistry, have transformed our understanding of cell structure and the function of the biomolecules within the cells.
Since these advanced microscopy techniques depend on switching fluorophores on and off via photobleaching or saturation, they also require high-brightness lasers as illumination sources.
The performance of the super-resolution techniques, however, is restricted on a quantum level by the stochastic nature of light. The randomness in the absorption of the photons by the microscope detectors (such as photodiodes or CCD sensors) introduces the so-called shot noise, which poses fundamental limits on the observable signal-to-noise ratio of the photon detectors. Therefore, the shot noise constrains the sensitivity, resolution, and imaging speed in modern super-resolution microscopes.
How to Evade Sample Photodamage
A long-established solution to the shot noise problem is to further increase the illumination intensity. Improving the spatial resolution through high-intensity illumination of the sample, however, results in photobleaching (irreversible loss of fluorescence during imaging) and makes phototoxicity (harmful physical and chemical reactions resulting from the interaction between light and cellular components) the biggest concern when employing these techniques. Typically, modern super-resolution microscopy techniques already employ illumination sources with intensities several orders of magnitude higher (typically up to 109 Wcm−2) than the light sources used in conventional microscopy (typically several Wcm−2).
Fundamentally, any measurement process, such as photon detection, is governed by the laws of quantum mechanics, which impose constraints on the precision of the measurement. Nevertheless, quantum mechanics provides possibilities for optical measurements with improved performance based on phenomena that harness the quantum effects to their advantage.
A team of researchers from Australia and Germany led by Prof. Warwick Bowen, head of the Quantum Optics Laboratory at the University of Queensland in Australia, recently developed a quantum-enhanced Raman microscopy technique that uses quantum-correlated illumination and overcomes problems associated with light-induced damage to the sample.
Raman microscopy is a nonlinear optical imaging technique that probes the vibrational spectra (associated with the oscillation of the intra-molecular chemical bonds) of complex molecules (such as biomolecules) and enables the imaging of chemical bonds with exceptionally high specificity without the need for fluorescence labeling. The technique usually employs two light sources: a pump laser (that interacts with the sample) and a longer wavelength probe (or Stokes) laser that stimulates the sample to re-emit light, boosting the signal strength.
Quantum Phenomena in Real-World Applications
The sensitivity of such stimulated (or non-linear) Raman microscope is directly related to the signal-to-noise ratio of the detected light. To increase the signal-to-noise ratio beyond the limit imposed due to possible photodamage of the sample, the researchers developed a particular type of photonic circuit called optical parametric amplifier that produces light in an exotic quantum state called squeezed light.
In this quantum state, the probe photons are no longer fully independent but correlate to the photons in the pump laser beam. Therefore, the fluctuations in the number of photons on the microscope detector no longer follow the statistical distribution observed in classical Raman microscopes. Importantly, the quantum-correlated illumination and detection allowed the reduction of the measurement noise floor below the shot noise.
One of the key challenges for the researchers was to create quantum entanglement that can produce bright enough light to be used as a light source in a microscope. By confining the photons into ultra-short laser pulses (a few billionths of a second long), Prof. Bowen and his colleagues produced entanglement that was 1012 times brighter than any previously used quantum-entangled light sources.
Future Applications of the Quantum Sensing Technology
When used to image the concentration of a specific molecular bond within a living yeast cell, the novel quantum-enhanced microscope demonstrated both sub-diffraction limited resolution and a 35% improvement of the signal-to-noise ratio without the need for excessively bright laser illumination.
Prof. Bowen's team achievement demonstrates that quantum correlations can provide a substantial performance advantage in microscopy. The researchers are aiming to achieve even higher levels of quantum correlation in their pulsed light source, enabling more than ten-fold signal-to-noise enhancement in the future.
References and Further Reading
Casacio, C.A. et al. (2021) Quantum-enhanced nonlinear microscopy. Nature 594, 201–206. Available at: https://doi.org/10.1038/s41586-021-03528-w
E. O. Potma (2021). Squeezed light improves sensitivity of microscopy technique [Online] www.nature.com Available at: https://www.nature.com/articles/d41586-021-01514-w (Accessed on 8 July 2021).
L. Crane (2021). Quantum microscope can examine cells in unprecedented detail. [Online] www.newscientist.com Available at: www.newscientist.com/article/2280216-quantum-microscope-can-examine-cells-in-unprecedented-detail (Accessed on 8 July 2021).
M. Frankel (2021). A quantum hack for microscopes can reveal the undiscovered details of life. [Online] www.theconversation.com Available at: https://theconversation.com/a-quantum-hack-for-microscopes-can-reveal-the-undiscovered-details-of-life-161182 (Accessed on 8 July 2021).
Lawrie, B. J. et al. (2019) Quantum Sensing with Squeezed Light. ACS Photonics 6 (6), 1307-1318. Available at: https://doi.org/10.1021/acsphotonics.9b00250
Disclaimer: The views expressed here are those of the author expressed in their private capacity and do not necessarily represent the views of AZoM.com Limited T/A AZoNetwork the owner and operator of this website. This disclaimer forms part of the Terms and conditions of use of this website.