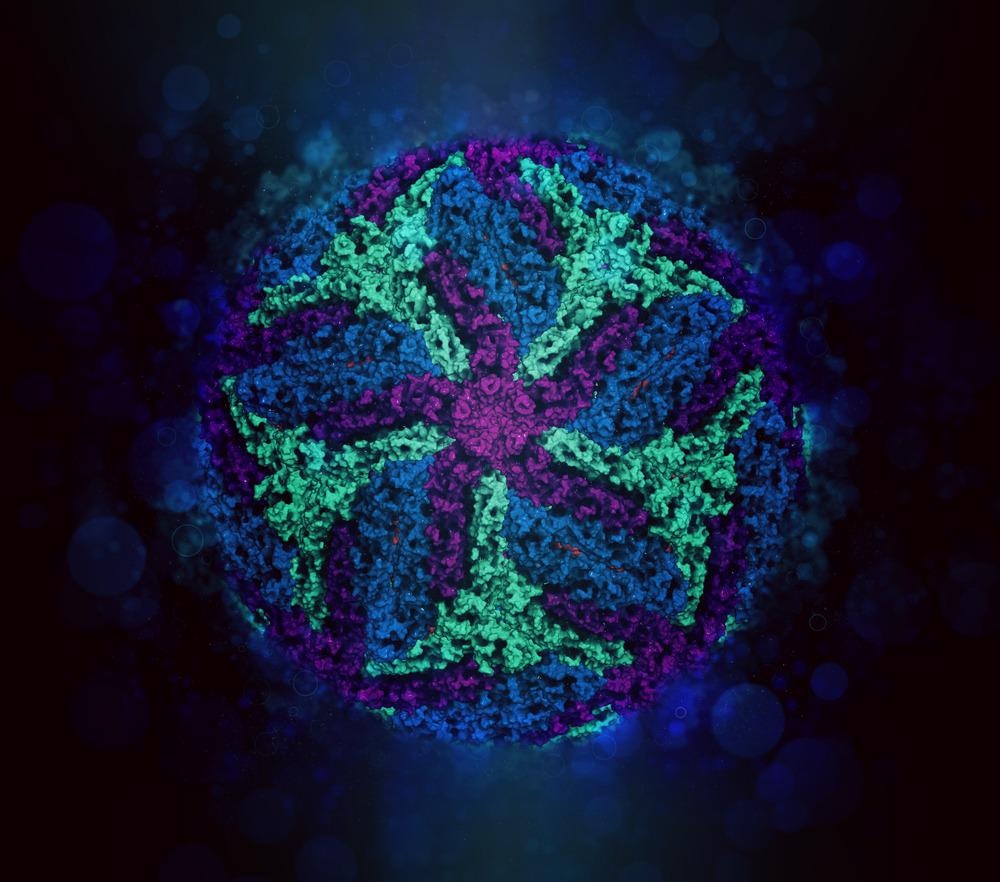
Image Credit: StudioMolekuul/Shutterstock.com
The impact of cryogenic electron microscopy, sometimes called cryo-EM, on life science was recognized with the 2017 Nobel Prize to Jacques Dubochet, Joachim Frank, and Richard Henderson for their contributions towards the development of cryo-EM for the high-resolution structure determination of biomolecules in solution.1 Cryo-EM has made it possible to record 3D structures of proteins with atomic resolution, even for samples that cannot be crystallized. This is essential for other structurally sensitive techniques such as X-ray diffraction.
Knowing the structures of macromolecules such as proteins and viruses is key to understanding their function and creating either new biomimetics, copying that function, or developing treatments for pathogens. To take a cryo-EM image, the sample is rapidly frozen under cryogenic conditions, and then the sample is bombarded with the beam of electrons that do the imaging. There are several ways to detect the electrons and construct the images from which the structural information is recovered.
Detection Schemes
Cryo-EM is a type of transmission electron microscopy (TEM) and uses similar detection techniques to TEM. Recently, detector technologies have meant that direct electron imaging has become one of the most popular implementations for electron detection. It allows for high spatial resolution and faster wide-field image collection.2
Direct detection methods remove the detector's need to include a scintillator and screen to convert the electron hits into bursts of light that optical cameras can record. Direct electron detection methods for cryo-EM and other electron spectroscopies generally enhance detection efficiencies and image resolution versus scintillator-based schemes.3
One of the challenges that cryo-EM faces as a technique is dealing with the inherently poor signal-to-noise due to the low electron absorption cross-sections of most light elements.4 This makes it challenging to obtain high contrast images, and image acquisition times can become impractically long. Sample damage may also become a concern if exposure times are too high.5
Event-Based Detection
A successful cryo-EM experiment, therefore, depends heavily on high-quality, efficient detection schemes. A well-chosen detector is essential to achieving the image resolution necessary to distinguish the structures of interest. Low-noise detectors with good sensitivities can also help improve the image contrast.
One alternative approach to traditional detection methods is to use event-based detection, which Direct Electron have implemented in their latest electron camera, the Apollo.6
Event-based detection works by moving much of the heavy data processing and image reconstruction steps onto the chip using edge computing with FGPAs. Rather than integrating the entire sensor and later analyzing for electron hits, which is the current standard procedure, the Apollo can process successful hits to generate super-resolution (67-megapixel frames) at high speeds before the data even leaves the camera.
Apollo – High Throughput Cryo-EM
The Apollo is the first event-based camera for cryo-EM. The incredibly rapid data processing has several advantages for the experiment, aside from the reduced data processing times. The event-based scheme means that it is possible to work with 10 times the electron beam brightness leading to a 10 times reduction in the exposure time compared to other direct detection methods.
Utilizing the latest in CMOS technologies, the Apollo has been designed for high throughput cryo-EM. The Apollo detector has been integrated with supporting software packages from Direct Electron, including SerialEM for microscopy automatization services. This, combined with the improved signal-to-noise and the Apollo’s compatibility with a wide range of dose rates, will dramatically reduce the experimental time to find optimal conditions for specific sample imaging.
Improvements in the ease-of-use and the flexibility that come with the Apollo will lead to a remarkable enhancement in cryo-EM productivity and open up new possibilities for addressing long-standing questions in structural biology.
As cryo-EM is still a very costly technique, with experiments often being located in user facilities, maximizing what measurements can be achieved in a short time will be invaluable for providing opportunities to screen and test new systems and optimize data outputs.
After eight years in development, Direct Electron has taken great care to design the Apollo so that it is compatible with an expansive range of TEM columns that can also be customized for individual experiments.
The ability to optimize experiments more carefully for individual sample types combined with unparalleled improvements in the low-noise CMOS chip will significantly impact increasing the number of sample types, particularly very poor electron absorbers, that can be measured with cryo-EM.
References and Further Reading
- Nobel Foundation (2017) The Nobel Prize in Chemistry 2017, https://www.nobelprize.org/prizes/chemistry/2017/summary/, accessed 20th March 2021
- McMullan, G., Faruqi, A. R., & Henderson, R. (2016). Direct Electron Detectors. Methods in Enzymology (1st ed., Vol. 579). Elsevier Inc. https://doi.org/10.1016/bs.mie.2016.05.056
- Hart, J. L., Lang, A. C., Leff, A. C., Longo, P., Trevor, C., Twesten, R. D., & Taheri, M. L. (2017). Direct Detection Electron Energy-Loss Spectroscopy: A Method to Push the Limits of Resolution and Sensitivity. Scientific Reports, 7(1), 1–14. https://doi.org/10.1038/s41598-017-07709-4
- Lyumkis, D. (2019). Challenges and opportunities in cryo-EM single-particle analysis. Journal of Biological Chemistry, 294(13), 5181–5197. https://doi.org/10.1074/jbc.REV118.005602
- Karuppasamy, M., Karimi Nejadasl, F., Vulovic, M., Koster, A. J., & Ravelli, R. B. G. (2011). Radiation damage in single-particle cryo-electron microscopy: Effects of dose and dose rate. Journal of Synchrotron Radiation, 18(3), 398–412. https://doi.org/10.1107/S090904951100820X
- Direct Electron (2021) Apollo, https://directelectron.com/, accessed 20th March 2021
Disclaimer: The views expressed here are those of the author expressed in their private capacity and do not necessarily represent the views of AZoM.com Limited T/A AZoNetwork the owner and operator of this website. This disclaimer forms part of the Terms and conditions of use of this website.