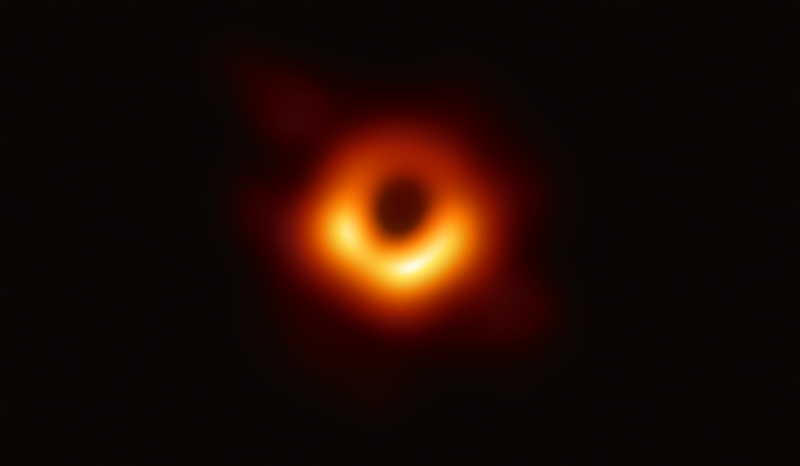
Image Credit: NASA/Shutterstock.com
On the anniversary of the first image of a black hole, new research has revealed details that were hidden within the picture and suggests what the future for black hole imaging could look like.
In April 2019 scientists revealed the first-ever image of the immediate environment around a black hole. The picture taken by the Event Horizon Telescope (EHT), became one of the most widely shared on social media that year, and the researchers who made the achievement possible were rightly lauded and celebrated.
This was not the end of the story for the image. Investigations were already underway to probe deeper beneath the surface of the pictured black hole that sits at the center of the galaxy M87 located 54 light-years from Earth.
One year after the proud announcement of one of humankind’s most awe-inspiring achievements, research has demonstrated how the black hole images can be used to reveal more about their subject than previously believed possible, and, in turn, teach us how to build a better black hole image.
Conducting the study required an eclectic mix of scientists from different fields, including astrophysics, cosmology, and computer science to calculate the intricate ring substructure within the golden ring that dominates the image of the black hole at the center of M87. The paper published in the journal Science Advances describes the substructure that is created by a phenomenon predicted in Einstein’s theory of general relativity known as gravitational lensing.
How Astronomers Took The First Ever Image Of A Black Hole
Video Credit: Tech Insider/YouTube.com
Turning Light Rays into Light Rings
Gravitational lensing describes what occurs when an object of considerable mass creates a ‘dent’ in the very fabric of spacetime around it, with this indentation causing the path of light rays passing the object to be curved. The most common analogy used to describe this phenomenon is to describe space as a stretched-out rubber sheet. Roll a marble — analogous to a light-ray — across the sheet and it will travel in a straight line to the other side.
But what if we place a bowling ball on the sheet and then re-roll our marble?
Clearly, the dent caused by the bowling ball will cause the path of the marble to curve. If this dent is extreme enough, rather than travel to the other side of the sheet, the marble will find itself trapped in a path curving around the bowling ball.
Marbles that pass too close to the bowling ball are pulled in and unable to escape. This is analogous to photons that pass beyond the ‘event horizon’ of the black hole — the point where nothing, not even light, has the necessary escape velocity to pull away from the massive gravitational influence of the black hole.
Due to photons being unable to escape the event horizon of the black hole, it casts a shadow on the bright emission of the gas and dust that surrounds it and feeds onto its surface. Around this shadow is a ring of photons produced by the strong gravitational effect outside the event horizon in the vicinity of the black hole.
Returning to the rubber sheet analogy, the marbles at this point will be trapped in a circular orbit around the bowling ball, just as photons caught orbiting the black hole outside the event horizon create a ‘photon ring.’
This ring is actually comprised of an infinite nested hierarchy of sub-rings; these become progressively brighter because the photons in the sharpest rings have made more orbits of the black hole before being recorded by the EHT.
According to general relativity, this photon ring should contain information about the limited characteristics a black hole is able to hold. These characteristics are limited because the ‘No-Hair Theorem’ of black holes says that such objects can only have the following qualities: mass, charge, and angular momentum (spin). This information should lie within the image of M87’s black hole.
Using Interferometers to Determine Photon Ring Substructure
The problem is that the image of these nested rings cannot be resolved in the EHT picture by the naked eye. Fortunately, arrays of telescopes called interferometers may be able to clearly determine this substructure and thus produce images in which features can clearly be determined.
Capturing black hole images requires many spatially distributed telescopes — an array that comprises the EH. However, the subrings could be resolved by using two telescopes that are very far apart.
The task of determining this photon ring substructure could be achieved easily by adding another space telescope to the observational power of the network of telescopes that comprises the Event Horizon Telescope. This would significantly boost the resolution of the Very Long Baseline Interferometry technique employed to capture the image of the M87 black hole.
However, creating a black hole image requires another type of power, aside from observational power. Considerable computing power is needed to reconstruct the data captured by the EHT and turn it into an image that makes sense to the human eye.
Computing the Black Hole with Continuous High-Resolution Image Reconstruction
To process the data captured by the eight telescopes of the EHT and turn it into the single cohesive image that was released to the public in April 2019, researchers, including computer scientist Katie Bouman, used a computer algorithm named CHIRP (Continuous High-resolution Image Reconstruction using Patch priors).
CHIRP was developed by a team of scientists from MIT’s Computer Science and Artificial Intelligence Laboratory, the Harvard-Smithsonian Center for Astrophysics and the MIT Haystack Observatory, with the aim of turning Earth into a giant radio telescope.
To do this and create an image such as that of M87’s black hole, the program has to filter through a huge amount of data from the telescopes to filter out atmospheric noise and other sources of interference.
Creating an even more detailed version of the image - one in which the sub-ring structure can be determined to unlock the information held within it - requires the development of an even more powerful computer program. Failing that, the researchers could use a program that could recreate simulated black hole images at a higher resolution than previously dreamed possible.
This was the task undertaken by George Wong, a physics graduate student at the University of Illinois at Urbana-Champaign. Wong’s software produced images that were decomposed into the predicted series of ultra-sharp sub-images.
Consider that each sub-ring is comprised of photons that represent the view of the universe from the face of the black hole. By collecting and resolving these photons, researchers are in a way recreating the cosmos as the black hole at the center of M87 has seen throughout its existence.
Experimental Black Hole Science
To theoretical physicists, the image of M87 was a significant step in validating decades of their work. The fact that the black hole so closely represented predictions made from the numerical solutions of general relativity helped confirm that our view of the Universe and the processes that govern its most massive objects are correct.
Images produced with a greater resolution that reveal the photon ring substructure will allow us to delve further into objects that, until last year, were almost purely theoretical. The age of experimental black hole science, as this new research shows, is finally upon us.
Click here for more information on photonics equipment
References and Further Reading
Johnson. M.D, Lupsasca. A, Strominger. A, et al. Universal interferometric signatures of a black hole’s photon ring (2020) Science Advances.
Disclaimer: The views expressed here are those of the author expressed in their private capacity and do not necessarily represent the views of AZoM.com Limited T/A AZoNetwork the owner and operator of this website. This disclaimer forms part of the Terms and conditions of use of this website.