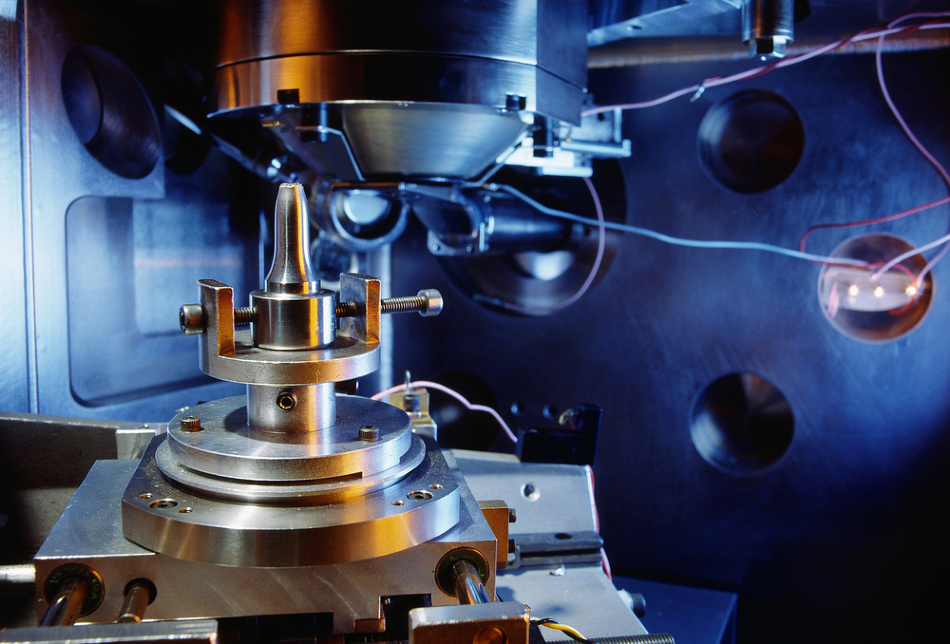
Image Credit: Bildagentur Zoonar GmbH/Shutterstock.com
Traditionally, when we think of microscopy, we think of optical microscopy that uses light to image objects. In the simplest form of optical microscope, lenses are used to focus the source light that is transmitted through the specimen of interest. This means an image is formed with greater magnification than the real object as the lens refracts in the incident light and converges it at a new location.
More complex microscopes use combinations of lenses and optics to allow for greater magnification and higher resolution imaging. Great care is also taken when designing microscopes to reduce the number of optical aberrations, which can result in a blurred or distorted image.
Aberrations occur when the light is spread out over a region of space, rather than focused on a single point. All lenses show some degree of non-ideal behavior but, depending on the defects, particular types of aberration may become more problematic.1 The degree of irregularity also affects the ultimate resolving power and meaningful magnification achievable by a microscope.
While different kinds of aberration usually contribute to the resolution limit of an instrument, even a perfect optical microscope with defect-free lenses is subject to the diffraction limit. The diffraction limit is the ultimate resolution an imaging system can achieve, defining the smallest distance between two points on an object that can still be seen as distinct objects.
As the diffraction limit is incident photon wavelength-dependent, to achieve better resolution microscopy images, one alternative has been to use UV or X-ray radiation instead of visible light. Another option is to use electrons, rather than light photons, to image the sample.
Optical Transfer Theory
One advantage of working with electrons over photons is that the greater mass of the electron means that even slower-moving particles have much shorter wavelengths. This results in a smaller diffraction limit and it is much easier to achieve higher imaging resolution with an electron beam as well as significantly higher magnifications.2
While the physical optics for steering and focusing electron beams are based on shaped magnetic fields rather than transparent glasses, many of the theories we know from visible optics can be applied to electron imaging as well.3
The transfer theory describes how an electromagnetic (or acoustic) wave moves through an object. In the context of optical transfer theory, this describes how the system handles the different spatial frequencies of the light. For example, the optical transfer theory can be used to explain how the final image displayed by a large projector on a wall will look in terms of resolution and contrast.
Click here for more information on photonics equipment
Any object will have an optical transfer function to describe its resolution and contrast or modulation. Often objects will instead be characterized by their modulation transfer function instead of the full optical transfer function.
The modulation transfer function is obtained from the full optical transfer function by merely taking its modulus.4 However, this does neglect the phase information that would be present in the optical version. This is unnecessary for many applications.
For Electrons
Optical transfer theory can be adapted for use with electron beams instead of photons.3 Much of the physics of imaging an object is unchanged whether using electrons or photons, though the significant difference in energies will affect the overall resolution of the final image. As electrons are charged particles, they will repel each other, changing the electron beam’s focus.
As well as resolution, modulation transfer functions often try to describe the ‘contrast’ of an imaging system. Typically, contrast is defined as the difference in the irradiation values at two points in an image, or how light or dark different areas appear. However, the modulation transfer more accounts explicitly for the point spread function, which is a measure of how spread out an image of a single point object becomes.
There are many ways of imaging objects using electron microscopy, including scanning electron microscopy (SEM) and transmission electron microscopy (TEM). The critical difference between these is whether the electrons are scattered from the sample and collected (SEM) or passed through the sample (TEM). This means that the image formation process occurs in slightly different ways.
Scattered electrons behave similarly to scattered light5. In SEM, the detector is placed to capture secondary electrons, electrons that have lost energy through inelastic collisions with the surface, as well as backscattered electrons from the electron beam that have undergone energy loss from elastic collisions with the sample. The incident beam is rastered over the sample to map out its structural shape.
The modulation transfer function of the detector will contribute to the overall resolution and imaging contrast of the instrument. TEM works similarly to an optical microscope, where the electrons are focused through a sample but then onto a fluorescent screen, which lights up when it is bombarded with electrons to produce an image that can be captured with a standard camera chip.
How is electron microscopy used in other areas of science?
References and Further Reading
- Booth, M. J. (2014). Adaptive optical microscopy: the ongoing quest for a perfect image. Light: Science and Applications, 3, e165. https://doi.org/10.1038/lsa.2014.46
- Koster, A. J., & Klumperman, J. (2003). Electron microscopy in cell biology: integrating structure and function. Nature Cell Biology, 6–10.
- Hanszen, K. J. (2018). The Optical Transfer Theory of the Electron Microscope: Fundamental Principles and Applications. Advances in Imaging and Electron Physics (Vol. 207). Elsevier Inc. https://doi.org/10.1016/bs.aiep.2018.04.001
- Williams, C. S. & Becklund, O. A., (2002). Introduction to the Optical Transfer Function, SPIE Press
- Davisson, C., & Germer, L. H. (1927). The Scattering of Electrons by a Single Crystal of Nickel. Nature, 119, 558–560.
Disclaimer: The views expressed here are those of the author expressed in their private capacity and do not necessarily represent the views of AZoM.com Limited T/A AZoNetwork the owner and operator of this website. This disclaimer forms part of the Terms and conditions of use of this website.