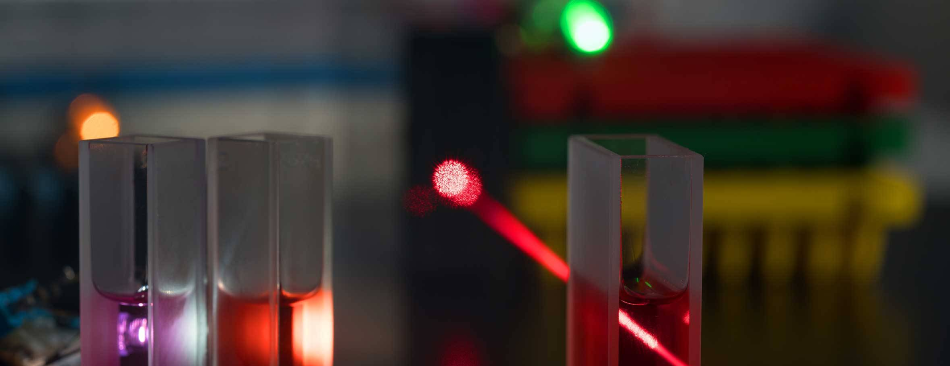
The category of instrumentation and applications that use laser sources is quickly developing. For example, flow cytometry, confocal and multiphoton microscopy, and Raman spectroscopy all make use of laser sources.
In all of these techniques, it is crucial to remove all undesirable laser scatter, plasma, and background to achieve optimum signal-to-noise.
Short pass and laser line edge filters can be utilized to ‘clean-up’ the signal at the source of the laser. Longpass edge and laser rejection filters can be employed for the rejection of unwanted noise at the detector.
A mixture of laser filter solutions can offer superior performance to holographic notch filters at a significantly reduced expense.
Every laser filter is configured with a high laser damage threshold of up to 1 watt/cm2.
Laser Line Filters
At the laser source, while the output is normally considered monochromatic and is outlined by a single output wavelength and a prominent line, lower level plasma, transitions, and ‘glows’ are often involved, all of which produce background errors.
Laser sources can additionally shift in wavelength according to the temperature, power, and even manufacturing limitations. The transmission of pure excitation energy needs a laser ‘clean-up’ filter to manage unwanted energy.
Centered on the resonance of the laser, laser line filters are narrow bandpass filters that decrease the secondary emissions and background plasma that frequently produce inaccurate signals.
When using LEDs and diode lasers, these filters can be utilized to enhance the monochrome of the light output. In the example of gas lasers, these filters can remove plasma in the deep blue wavelength area.
Laser line filters offer a 60 to 90% throughput (excluding UV) with spectral control from 0.85 to 1.15 of the CWL. An accessory blocker can be supplied to manage a much broader spectral range, from the deep UV to the IR. This extra blocker produces an insignificant loss of throughput (<20%).
Laser Edge Filters
Both the wanted signal and undesired scatter will be present at the detector, with the signal’s orders of magnitude being less than the scatter.
Scatter is produced by slight irregularities and features of the application and system optics, such as uncontrolled light from the holder and sample. In order to enhance signal-to-noise, both edge filters and laser rejection filters can be utilized to weaken or prevent, the scattered energy from contacting the detector.
Longpass edge filters are an ideal solution for laser rejection when employed in a collimated light path on the detector area of the system.
They diminish shorter wavelengths to approximately 0.7 l edge or the deep UV for l less than around 500nm and display steep slopes (less than 3% on the 5-Decade slope factor), high throughput of the Raman signal, and deep blocking of the laser line (an OD that is greater than 5).
Edge filters will transmit approximately 85% of Stokes or anti-Stokes fluorescence or Raman signal and detail very high contrast between the Raman and Rayleigh transmission. They are created with edges specified as 1.03 x laser wavelength.
For the most effective performance, angle tuning is necessary. The performance of edge filters is greater than holographic notch filters and low throughput monochromators at a significantly reduced cost.
Angle Tuning Edge Filters
Angle tuning can be used on all edge filters to attain optimal signal-to-noise. The transmission curve will blue-shift when the filter is angle tuned.
Angle tuning enables Raman signals that are near the laser line to pass through the filter, which causes blocking at the laser line. The filter can be configured up to around 15 degrees from normal incidence.
At a 15-degree angle of incidence, the cut-on wavelength of the longpass edge filter will shift blue by around 1% of the cut-on value at normal incidence.
A filter that cuts on at 600 nm with normal orientation will cut on at 594 nm when positioned to 15 degrees. A result of this blue shift is that the laser line blocking will reduce by around two optical densities.
An additional capability of angle tuning is that reflected energy at the optical axis is redirected. For longpass edge filters, a filter should be chosen to contain an edge that is to the red of the intended cut-off, and the filter angle should be changed until the required performance is attained.
A further option for attaining greater transmission at small Raman shifts is to utilize two filters in sequence, each configured to block the laser line at OD levels of 2 to 3.
When utilized simultaneously, there will be additive blocking at the laser line (an OD of 4 to 6), and the 5-decade slope factor is successfully reduced from 3% to as small as 1.5%.
Laser Rejection Filters
Both scatter and signal will be present at the detector, with the scatter orders of magnitude being greater than the signal. To optimize signal-to-noise, both edge filters and laser rejection filters can be employed to weaken, or stop, the scattered energy from contacting the detector.
Laser rejection filters are created to block over 99.9% of light in a bandwidth of 15 to 40 nm. The standard transmission rate outside of the top band is 75%, apart from in spectral areas where lower and higher harmonics result in comparably high reflection.
Rejection band filters that are specially designed can reflect multiple spectral bands and function at off-normal angles of incidence. Rejection, or notch, filters offer the ability to quantify both Stokes and anti-Stokes signals at the same time and have the tunability for a range of laser lines.
Edge filters can similarly be employed for the rejection of lasers, offering deeper blocking of the steeper edges and laser line, for smaller Stokes shifted applications.
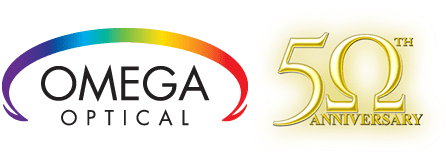
This information has been sourced, reviewed and adapted from materials provided by Omega Optical, Inc.
For more information on this source, please visit Omega Optical, Inc.