When using a hydrophone to identify a broadband acoustic signal, artifacts resulting from the convolution will manifest. This is especially important for bubble collapse shock waves as both an obviously negative phase and the peak positive pressure amplitude or incorrectly measured if the signal is not deconvolved.
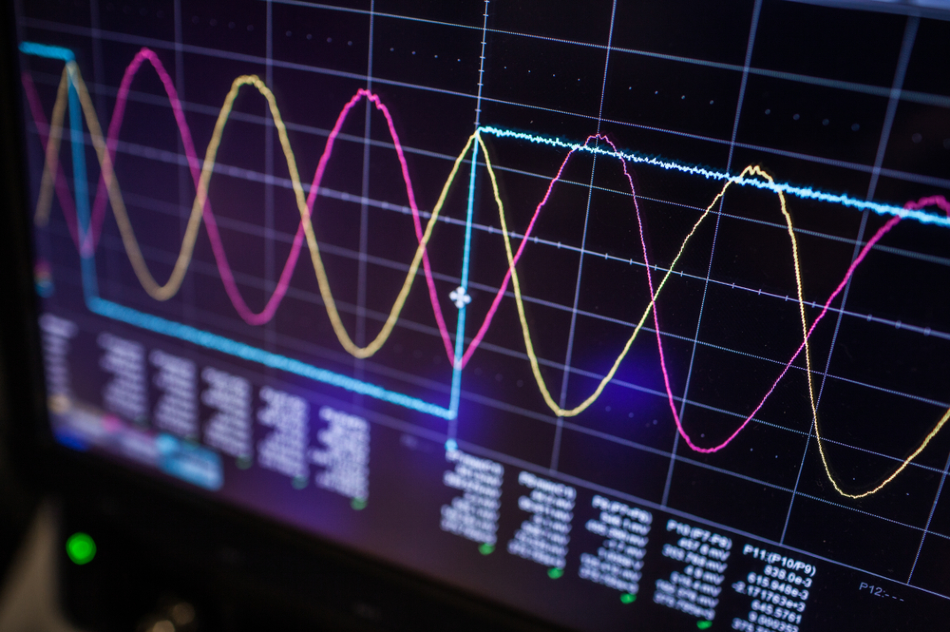
Image Credit: Shutterstock/lightpoet
The collapse of a laser induced bubble and the consequent generation of a shock wave is recorded in this experiment utilizing high speed imaging. The shock wave is identified by a needle hydrophone at three distances. The experimental results are compared to theoretical arguments regarding the properties of the shock wave.
The ability to perform unrivaled shadowgraphic imaging of the bubble collapse was critical in order to achieve the results, both for managing the size of the bubble and the spherical rebound after the collapse. The high speed observation was additionally employed to inform the simulation of the theoretical prediction.
Experimental Configuration
A single laser-induced bubble (LIB) is created when a laser pulse comprising of enough energy to cause an optical breakdown is focused into a liquid.
A LIB firstly undergoes a phase of expansion as a reaction to the energy deposition. The inaction of the host medium slows down this which eventually causes the bubble to contract and disintegrate, often followed by multiple rebound oscillations.
The acoustic detection of the LIB process is characterized by the generation of a sequence of shock waves.
The first is emitted by the plasma developed upon the absorption of the laser pulse, with a second generated throughout the collapse of the main bubble after a length of time that is the same as the oscillation time of the LIB. Subsequent rebounds may also generate shocks of decreasing pressure amplitudes.
.jpg)
Figure 1. Schematic of experimental setup.
To reliably analyze the BCSWs, LIBs are produced within a tailor-made chamber, displayed schematically in Figure 1. This measures 420 x 438 x 220 mm3 and is filled with deionized, degassed water.
Two of the walls are recessed which allows the imaging optics to be positioned in proximity to the desired location of the LIB, enabling a spatial resolution at 7.6 ± 0.1 μm per pixel.
One 4.0 ± 0.2 mJ (instrument error regarding the manufacturer), 6-8 ns laser pulse (Nano S 130-10 frequency doubled Q-switched Nd:YAG, Litron Lasers, UK) is brought to a focus through a long working distance microscope objective lens (50 x 0.42 NA Mitutoyo, Japan), immersed in a sealed unit, and positioned on an xyz manipulator (Velmex Motor, Bloomfield, NY, USA).
High-speed shadowgraphic imaging of the consequent cavitation activity is performed at 5 x 106 frames per second (HPV-X2, Shimadzu, Japan), with simultaneous 10 ns laser pulses (CAVILUX Smart, Cavitar, Finland) offering successful temporal resolution and illumination.
A delay generator (DG535, Stanford Research Systems, USA) enables electronic triggering to coincide with every instrument.
The HPV-X2 camera provides 256 frames per image sequence so that the dynamics of the collapse of a single bubble is adequately sampled for the purposes of modeling.
The majority of the literature on this kind of experiment is reliant upon the choice of frames from several different high-speed sequences of a range of different bubbles, under the premise that every bubble attains a similar maximum radius and undergoes a similar collapse.
Shockwave created by bubble collapse illuminated with CAVILUX laser light
Figure 2. Video of shockwave created by bubble collapse.
.jpg)
Figure 3. High-speed images of a collapsing laser induced bubble with the emission of a shock wave, and a spherical rebound. Imaging is undertaken at 5 million frames-per-second with synchronous 10 ns laser pulses CAVILUX Smart providing the illumination and effective temporal resolution.
Results After Deconvolution of Needle Hydrophone
When deconvolution is used to remove the impulse response from the needle hydrophone, the experimental measurements are in reasonable agreement with the theoretical competition.
This is especially apparent because the clear negative phase in the convoluted experimental measurement has disappeared.
For broadband signals, it is critical to ensure that the measurement is deconvolved with data concerning both the phase and magnitude, so that the temporal shape of the measurement remains physical.
.jpg)
Figure 4. Theoretical computation (stapled black) and deconvolved experimental measurement (solid black) of bubble collapse shock wave at distances 30, 40, and 50 mm from the nucleation site.
References and Further Reading
Characterizing focused ultrasound via high speed shadow graphic imaging at 10 million frames per second, Kristoffer Johansen, Jae Hee Song and Paul Prentice, Ultrasonics Symposium (IUS), 2016 IEEE International, Date of Conference: 18-21 Sept. 2016
An analysis of the acoustic cavitation noise spectrum: The role of periodic shock waves, Jae Hee Song, Kristoffer Johansen, Paul Prentice (CavLab, Cluster of Ultrasound Science, Technology and Engineering Research, University of Glasgow, UK); JASA, Volume 140, Pages 2494 – 2505, 2016
Acknowledgments
Produced from materials originally authored by Kristoffer Johansen, Jae Hee Song, Keith Johnston, and Paul Prentice from the University of Glasgow.
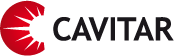
This information has been sourced, reviewed and adapted from materials provided by Cavitar.
For more information on this source, please visit Cavitar.