Raman spectroscopy is a powerful and versatile analytical technique used to study molecular and material samples. The technique is based on the inelastic scattering of light, also known as Raman scattering, which allows for the identification and quantification of chemical bonds in a sample.
Each molecule or material has a unique set of chemical bonds, and the frequency at which these bonds vibrate depends on the local chemical environment. This gives each molecule a distinct Raman "fingerprint" that can be used for identification.
The intensity of the observed transitions can also be used to obtain quantitative information about a sample. A typical Raman spectroscopy instrument will consist of a light source, beam handling optics, and a detector.
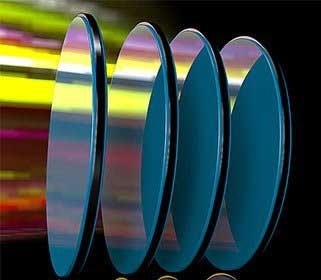
Image Credit: Iridian Spectral Technologies
Understanding Raman Signals
The most common method of Raman spectroscopy involves monitoring chemical vibrations by inducing a vibrational transition in the molecule with light. When light strikes a sample, some photons are elastically or inelastically scattered.
Because there is no energy transfer between the incident photon and the specimen in elastic scattering, the measured light will have the same wavelength as the input light. There is an energy transfer between the sample and the photon during an inelastic scattering event.
Stokes scattering refers to light that has lost energy owing to sample interaction, whereas anti-Stokes scattering refers to light that has gained energy. The energy loss or gain in the Stokes or anti-Stokes spectrum is related to the molecule's vibrational structure. This is what can be used to identify species.
Stokes lines are typically more intense than anti-Stokes lines because they relate to interactions with molecules in the ground state. Most studies conducted at room temperature will have a substantially higher proportion of molecules in the ground state.1
Raman Spectroscopy Principles and Instrumentation
One of the difficulties in measuring Raman spectroscopy is that the Raman process is not the only one that may be initiated by light. If a molecule is electrically stimulated by a light source, it may fluoresce, indicating energy relaxation via photon emission.
The fluorescence background is a serious concern in Raman spectroscopy because these background signals have a much wider energy range and are more powerful than inelastically scattered Raman signals. Raman signals are naturally weak, with intensity scaling with 1/λ4, where λ is the excitation wavelength used to initiate the Raman process.
Using shorter wavelength excitation sources in Raman spectroscopy increases the strength of the Raman but also the likelihood that there will be enough energy to activate an electronic transition in the sample and initiate a fluorescence process.
To fully leverage the power of Raman spectroscopy for sample identification, careful spectrometer layout for Raman spectroscopy to minimize these competing contributions is essential.
Longer wavelength excitation and the use of more sensitive Fourier Transform Raman spectroscopy instruments, as well as surface-enhanced approaches to boost Raman signal levels, have become widespread in recent years.
Optics such as lenses are employed in Raman spectroscopy to focus beams and boost local excitation energy, while high transmission optics are used to assure minimum signal-collecting losses.
Optical filters are another important tool for suppressing undesired signals, whether they be residual light from the excitation source or unwanted scattering. In Raman spectroscopy, the appropriate filter selection can help enhance signal-to-noise, remove undesired backgrounds, and even improve instrument resolution.
High-performance filters are critical for attaining optimal performance in Raman spectroscopy instruments, especially when dealing with difficult samples with low signal levels or when very high resolution is required.
References and Further Reading
- Kauffmann, T. H., Kokanyan, N., & Fontana, M. D. (2018). Use of Stokes and anti‐Stokes Raman scattering for new applications. Journal of Raman Spectroscopy, 50, 418–424. https://doi/10.1002/jrs.5523
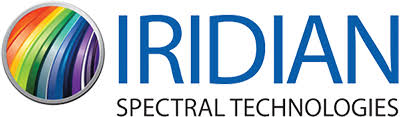
This information has been sourced, reviewed and adapted from materials provided by Iridian Spectral Technologies.
For more information on this source, please visit Iridian Spectral Technologies.